Hitting the brake: miR-31 regulates CD8 T cell effector function
Cytotoxic CD8 T cells have pivotal effector and regulatory immune-functions and are, as such, involved in the host-immune response following infection. Dysfunction of CD8 T cells, on the other hand, may contribute to autoimmunity. CD8 T cells exert their function by releasing cytotoxic mediators and cytokines. Acute infection triggers a response to effectively clear the pathogen and develop long-lasting protective memory, while chronic infections may lead to exhaustion and dysfunction of CD8 T cells. The latter phenomenon relates to the tunable balance of the immune system to preserve tolerance and prevent development of autoimmunity. CD8 T cells also play a crucial role in preventing development of cancer cells. Tumor cells may evade an effective anti-tumor immune response and clinical exploitation of immune checkpoints to break this tolerance and induce anti-tumor immunity clearly illustrates the two-edged characteristics of the immune response. Vice versa, autoimmune disorders and chronic infections may lead to the onset of cancer, which suggests that these pathological conditions may share mutual pathways. Understanding the molecular basis of autoreactive and defective CD8 T cell responses provides a basis for the rational design of immunotherapies aiming to restore CD8 T cell function and combat chronic viral infections and cancer.
Recently, Moffett et al. showed involvement of miR-31 in the inhibition of mouse CD8 T-cell effector response resulting in immune exhaustion during the chronic stage of in vivo lymphocytic choriomeningitis viral infection (LCMV) in a T cell intrinsic manner (1). In naive CD8 T cells, a substantial upregulation of miR-31 was observed as early as 8 hrs following T-cell receptor (TCR)/CD3 and CD28 priming. MiR-31 upregulation involved the calcium—nuclear factor of activated T-cell (NFAT) signaling pathway and its expression levels remained high for 2 weeks in the presence of IL-2. Interestingly, miR-31 was shown previously to increase IL-2 production during T cell activation by directly targeting and inhibiting KSR2 expression. Moreover, miR-31 enhanced NFAT activity in Jurkat T cells (2,3). These findings suggest a positive regulatory loop between miR-31 and NFAT activity with miR-31 enhancing NFAT while NFAT may induce expression of miR-31 directly or indirectly through calcium signaling (Figure 1). MiR-31 is repressed by hypermethylation in breast cancer, while polycomb group protein enhancer of zeste homolog 2 (EZH2) suppressed miR-31 expression in colorectal cancer (4,5). Expression of miR-31 is tissue-dependent and its transcriptional regulation has been shown to be differentially affected in different types of cancer. Induction of miR-31 upon TCR activation as shown by Moffett et al. raises an interesting scenario in which TCR priming and costimulation via CD28 and IL-2 initiates a cascade of downstream signaling events which might involve changes in histone modification in a way that allows and stabilizes miR-31 transcription. Of note and in contrast to the findings of Moffett et al., we have consistently noted reduced expression of miR-31 in sorted primary CD8+ CD45RO negative naive human T cells following five days of stimulation with plate bound anti-CD3 and soluble anti-CD28 (6). Apart from (subtle) experimental differences, such as the interspecies-inherent different way by which naive T-cells are defined, we do not have an explanation for the reported contradictory regulation of miR-31 expression.
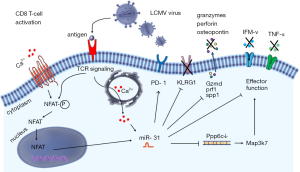
To identify miR-31 targets in CD8 T cells Moffett et al. overexpressed miR-31 in murine transgenic ovalbumin-specific OT-1 CD8 T cells and used microarray expression profiling to identify putative miR-31 target mRNAs (see Table 1). The authors identified 8 new targets of miR-31, as well as 4 previously reported miR-31 targets from in vivo studies in mice, i.e., Ppp6c, Lats2, Oxsr1 and Stk40, whereas Elavl1 was found to be regulated by miR-31 in human lung tissue. Some of these identified miR-31 targets are also known to be regulated by other miRNAs in human tissues, including microRNAs regulated by T cell activation, such as miR-17 and miR-20a (see Table 1) (6). Moffet et al. also noted increased expression of type I interferons (IFNα/β), and genes encoding interferon regulatory transcription factors (Irf3 and Irf7) upon miR-31 overexpression. IFNα/β has also been shown to be regulated by miR-155, which is induced upon human T-cell activation and differentiation. Ablation of miR-155 diminished viral clearance by CD8 T cells, whereas miR-31 inhibition enhanced viral clearance suggesting possible counterbalancing of T-cell effector function by these microRNAs (17).
Table 1
Validated direct miR-31 targets | Also regulated by | References |
---|---|---|
Cdkn1a* | miR130a, miR-17†, miR-20a†, miR-22 | (7-9) |
Coro7* | ||
Elavl1 | miR-9 | (10) |
Ifi30* | miR-BART1, miR-BART2, miR-BHRF1-2 | (11) |
Ilf3 | ||
Lats2 | miR-103, miR-107, miR-25 | (12,13) |
Oxsr1 | ||
Ppp6c | miR-373 | (14) |
Psd4* | ||
Rab1b* | miR-502 | (15) |
Sh2d1a* | ||
STK40 | miR-130a | (16) |
Stra13* |
*, genes not identified before as targets of miR-31; †, miRNAs up-regulated during T-cell activation.
IFNs are secreted by virus infected cells and promote antigen presentation and immune effector functions via modulating innate and adaptive immunity (18). Genes induced by IFNs contain an IFN stimulated response element (ISRE), which binds and activates IFN-regulatory factor (IRF) transcription factors such as IRF3 and IRF7. To investigate which genes and pathways are affected by miR-31 deletion in response to IFN-β signaling, Moffett et al. generated ‘miR-31−/− mice’ using mice with a floxed miR-31 gene locus and a Zp3 promoter driven Cre-recombinase. Hence, these mice have floxed gene excision in the female germline with whole-body inactivation of this miRNA (19). Ex vivo stimulation of CD8 T cells isolated from miR-31−/− mice via CD3/CD28 for 7 days revealed minor changes in expression of (phosphorylation of signal transducer and activator of transcription 1 (STAT1), STAT2, IRF9 and suppressor of cytokine signaling 1 (SOCS1), which are all downstream of type I interferon (IFN-I) signaling. Nevertheless, a clear increased abundance of multiple effector T cell signature molecules was observed in these mice. These data indicate that miR-31 dampens the effector function of CD8 T cells predominantly during secondary responses as observed in chronical viral infections.
IFN responses are tightly regulated. Several miRNAs such as miR-29, miR-155, miR-146a and miR-221/222 have been shown to regulate IFN signaling by affecting expression of cell surface IFN receptors, SOCS1 activity and transcription factors such as NFkB and STAT1/2 (20). Moffett et al. identified Ppp6c as a potential candidate involved in the regulation of IFN signaling in T cells. Short hairpin (sh)RNA mediated knockdown of Ppp6c increased the sensitivity of CD8+ T cells to type I IFNs in vitro, phenocopying the effect of miR-31 overexpression on these cells.
The physiological involvement of miR-31 in regulating the host immune response was addressed by assessing the course and severity of chronic LCMV infection in mice proficient and deficient for miR-31. Both wild type and miR-31−/− mice displayed signs of disease following viral infection. However, miR-31−/− mice performed better than the wild type mice in viral clearance as evidenced by higher body weights and improved disease resolution. The improved performance of miR-31−/− mice was associated with a higher percentage of tetramer positive, anti-viral CD8 T cells in miR-31-/- mice than in wild type mice. The percentage of memory T cell subsets was similar between control and experimental mice, whereas an increased percentage of killer cell lectin-like receptor subfamily G member 1 (KLRG1) positive T cells and a reduced percentage of exhausted programmed cell death 1 (PD1) positive T cells was observed by the authors. These findings support a role of miR-31 in dampening the T cell response during chronic infections.
Chronic viral infection exhausted CD8 T cells commonly show defective secretion of pro-inflammatory cytokines upon recall responses. Using wildtype and miR-31 knockout mice the authors showed that LCMV specific CD8 T cells from wild type mice secreted less IFN-γ and TNF-α when compared to LCMV specific CD8 T cells of miR-31−/− mice. However, depletion of miR-31 did not influence levels of secreted soluble IL-2. Although not consistent for all cytokines, these experiments show some pivotal regulatory effects of miR-31 in T cell exhaustion induced by chronic viral infection.
Moffett et al. demonstrate that miR-31 integrates different levels of T-cell function and as such regulates and possibly fine tunes the balance of effective T cell immunity versus tolerance. These findings open the possibility to target miR-31 as a therapeutic strategy to modulate T cell-immunity. Blocking miR-31 or regulating its expression might restore function of defective CD8 T cells in a T-cell intrinsic manner. This could possibly be applied in parallel with immune checkpoint inhibition, currently exploited therapeutically for the treatment of cancer. However, further studies will be needed to provide a true and integrated mechanistic understanding of the molecular and physiological pathways affected by miR-31, especially in man. Further elucidation of the way by which direct miR-31 targets affect downstream indirect targets of miR-31 in relation to the dysfunctional phenotype of CD8 T cells is required. This includes regulation of miR-31 expression in primary versus chronic viral infection. In this respect, it will be crucial to also address the apparent differences in the regulation and function of miR-31 between mice and man.
MiR-31 has been shown to regulate diverse cellular and developmental processes by targeting genes involved in cell proliferation, differentiation and cell death. The current study has extended this scope towards T cells, in which miR-31 seems to have a pleotropic, yet integrated role in the regulation of T-cell functionality.
Acknowledgments
Funding: None.
Footnote
Provenance and Peer Review: This article was commissioned and reviewed by the Section Editor Yikun Yao (Shanghai Institutes for Biological Sciences, Chinese Academy of Sciences, Shanghai, China).
Conflicts of Interest: All authors have completed the ICMJE uniform disclosure form (available at http://dx.doi.org/10.21037/ncri.2017.09.03). The authors have no conflicts of interest to declare.
Ethical Statement: The authors are accountable for all aspects of the work in ensuring that questions related to the accuracy or integrity of any part of the work are appropriately investigated and resolved.
Open Access Statement: This is an Open Access article distributed in accordance with the Creative Commons Attribution-NonCommercial-NoDerivs 4.0 International License (CC BY-NC-ND 4.0), which permits the non-commercial replication and distribution of the article with the strict proviso that no changes or edits are made and the original work is properly cited (including links to both the formal publication through the relevant DOI and the license). See: https://creativecommons.org/licenses/by-nc-nd/4.0/.
References
- Moffett HF, Cartwright AN, Kim HJ, et al. The microRNA miR-31 inhibits CD8+ T cell function in chronic viral infection. Nat Immunol 2017;18:791-9. [Crossref] [PubMed]
- Xue F, Li H, Zhang J, et al. miR-31 regulates interleukin 2 and kinase suppressor of ras 2 during T cell activation. Genes Immun 2013;14:127-31. [Crossref] [PubMed]
- Fan W, Liang D, Tang Y, et al. Identification of microRNA-31 as a novel regulator contributing to impaired interleukin-2 production in T cells from patients with systemic lupus erythematosus. Arthritis Rheum 2012;64:3715-25. [Crossref] [PubMed]
- Augoff K, McCue B, Plow EF, et al. miR-31 and its host gene lncRNA LOC554202 are regulated by promoter hypermethylation in triple-negative breast cancer. Mol Cancer 2012;11:5. [Crossref] [PubMed]
- Kurihara H, Maruyama R, Ishiguro K, et al. The relationship between EZH2 expression and microRNA-31 in colorectal cancer and the role in evolution of the serrated pathway. Oncotarget 2016;7:12704-17. [Crossref] [PubMed]
- Teteloshvili N, Smigielska-Czepiel K, Kroesen BJ, et al. T-cell Activation Induces Dynamic Changes in miRNA Expression Patterns in CD4 and CD8 T-cell Subsets. Microrna 2015;4:117-22. [Crossref] [PubMed]
- Brock M, Haider TJ, Vogel J, et al. The hypoxia-induced microRNA-130a controls pulmonary smooth muscle cell proliferation by directly targeting CDKN1A. Int J Biochem Cell Biol 2015;61:129-37. [Crossref] [PubMed]
- Tsuchiya N, Izumiya M, Ogata-Kawata H, et al. Tumor suppressor miR-22 determines p53-dependent cellular fate through post-transcriptional regulation of p21. Cancer Res 2011;71:4628-39. [Crossref] [PubMed]
- Trompeter HI, Abbad H, Iwaniuk KM, et al. MicroRNAs MiR-17, MiR-20a, and MiR-106b act in concert to modulate E2F activity on cell cycle arrest during neuronal lineage differentiation of USSC. PLoS One 2011;6:e16138 [Crossref] [PubMed]
- Jeyabal P, Thandavarayan RA, Joladarashi D, et al. MicroRNA-9 inhibits hyperglycemia-induced pyroptosis in human ventricular cardiomyocytes by targeting ELAVL1. Biochem Biophys Res Commun 2016;471:423-9. [Crossref] [PubMed]
- Tagawa T, Albanese M, Bouvet M, et al. Epstein-Barr viral miRNAs inhibit antiviral CD4+ T cell responses targeting IL-12 and peptide processing. J Exp Med 2016;213:2065-80. [Crossref] [PubMed]
- Zheng YB, Xiao K, Xiao GC, et al. MicroRNA-103 promotes tumor growth and metastasis in colorectal cancer by directly targeting LATS2. Oncol Lett 2016;12:2194-200. [PubMed]
- Zhang M, Wang X, Li W, et al. miR-107 and miR-25 simultaneously target LATS2 and regulate proliferation and invasion of gastric adenocarcinoma (GAC) cells. Biochem Biophys Res Commun 2015;460:806-12. [Crossref] [PubMed]
- Wu N, Liu X, Xu X, et al. MicroRNA-373, a new regulator of protein phosphatase 6, functions as an oncogene in hepatocellular carcinoma. FEBS J 2011;278:2044-54. [Crossref] [PubMed]
- Zhai H, Song B, Xu X, et al. Inhibition of autophagy and tumor growth in colon cancer by miR-502. Oncogene 2013;32:1570-9. [Crossref] [PubMed]
- Xiong Y, Chen H, Liu L, et al. microRNA-130a Promotes Human Keratinocyte Viability and Migration and Inhibits Apoptosis Through Direct Regulation of STK40-Mediated NF-kappaB Pathway and Indirect Regulation of SOX9-Meditated JNK/MAPK Pathway: A Potential Role in Psoriasis. DNA Cell Biol 2017;36:219-26. [Crossref] [PubMed]
- Gracias DT, Stelekati E, Hope JL, et al. The microRNA miR-155 controls CD8(+) T cell responses by regulating interferon signaling. Nat Immunol 2013;14:593-602. [Crossref] [PubMed]
- Ivashkiv LB, Donlin LT. Regulation of type I interferon responses. Nat Rev Immunol 2014;14:36-49. [Crossref] [PubMed]
- Lewandoski M, Wassarman KM, Martin GR. Zp3-cre, a transgenic mouse line for the activation or inactivation of loxP-flanked target genes specifically in the female germ line. Curr Biol 1997;7:148-51. [Crossref] [PubMed]
- David M. Interferons and microRNAs. J Interferon Cytokine Res 2010;30:825-8. [Crossref] [PubMed]
Cite this article as: Teteloshvili N, Kluiver J, van den Berg A, Kroesen BJ. Hitting the brake: miR-31 regulates CD8 T cell effector function. Non-coding RNA Investig 2017;1:8.