Enabling EMT: N-BLR promotes metastasis in colorectal cancer via regulation of epithelial-to-mesenchymal transition
Over the past twenty years, the advent of deep sequencing technology has revealed the pervasive and widespread nature of transcription across the human genome, with ~80% being transcribed at some point during development (1). Given that <2% of the human genome is composed of protein-coding genes, there exists a vast and expanding network of non-coding RNAs (ncRNAs), including long non-coding RNAs (lncRNAs), the majority of which remain functionally uncharacterized. However, members of this diverse and broad class of large (>200 nt) ncRNAs have emerged as major regulators of several key biological processes, including differentiation and oncogenesis (2,3).
LncRNAs form a class of large RNAs with no apparent protein-coding function. The highly tissue-specific expression of lncRNAs (4-6), combined with their dysregulation in several diseases (7,8), supports their role as master regulators of gene expression. They can regulate expression through a variety of epigenetic mechanisms and signal transduction pathways, acting in the nucleus or cytoplasm. In particular, recent studies have revealed that lncRNAs can function as competitive endogenous RNA (ceRNA), binding to and sequestering microRNAs (miRNAs), thus relieving post-transcriptional repression of miRNA targets (9,10). Growing evidence for this novel mechanism of miRNA regulation has sparked an increase in publicly available databases (11,12) of both experimentally supported (e.g., CLIP-seq) and computationally predicted ceRNA regulatory networks. While these curated databases are good starting points for identifying potential miRNA-lncRNA binding sites, they represent a broad overview of putative interactions that may not be physiologically relevant due to stoichiometric differences within different cell types. For example, mRNA/miRNA expression levels tend to be significantly higher than levels of potential miRNA sponges (e.g., lncRNAs, circRNAs), challenging the hypothesis that lowly-expressed ceRNAs can sufficiently sequester miRNAs to relieve post-transcriptional repression of target mRNAs (13). Despite these controversies surrounding the ceRNA hypothesis, several examples exist of ncRNAs functionally acting as miRNA sponges in the context of cancer, regulating proliferation (14), apoptosis (15), and epithelial-to-mesenchymal transition (EMT) (16).
The recent study by Rigoutsos et al. (17) describes another example of an lncRNA functioning as a miRNA sponge, using in vitro and in vivo methods to elucidate its role in promoting EMT in colorectal cancer (CRC) by sequestering members of the miR-200 family (Figure 1). Specifically, the authors demonstrate that targeting of mRNAs by miR-141/200c-3p is mediated by a pyknon motif within N-BLR that overlaps with a miR-200c-3p binding site, resulting in N-BLR-mediated derepression of regulators of apoptosis (XIAP) as well as EMT (ZEB1).
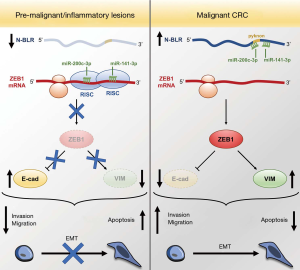
Recently, several ncRNA elements have been identified as playing essential roles in CRC (16,18,19). Rigoutsos et al. began their search for novel ncRNA regulatory loci in CRC by investigating expression of pyknon-containing DNA regions. Pyknons are short, organism-specific DNA sequence motifs that are repeated throughout intergenic regions and introns, as well as within at least one mRNA. The abundance of pyknons within the genome suggests a potential role in regulating expression of numerous non-coding transcripts, and one study found that DNMT1 RNA-binding was enriched at hypo-methylated pyknon-containing sequences (20).
RT-qPCR profiling of a panel of 11 pyknon regions within functionally relevant sequences revealed dynamic expression across CRC lines, tissue samples, and patient-derived xenografts (PDXs). Specifically, the authors observed one pyknon, pyk-reg-90, was significantly correlated with high tumor stage, poor overall survival, and was enriched in metastatic CRC PDXs, providing the first clue to its ability to regulate invasion and migration of CRC. GeneRacer cloning revealed that pyk-reg-90 resided within a novel, primate-specific 844-nt mono-exonic lncRNA that they termed “N-BLR”. After confirming the non-coding nature and cytoplasmic localization of N-BLR, they performed in situ hybridization (ISH) on colon tissue microarrays and observed higher expression levels in epithelial malignant cells compared to premalignant or inflammatory lesions.
Following their initial discovery of N-BLR enrichment in malignant tumors, Rigoutsos and colleagues investigated the function of N-BLR through a series of si/shRNA knockdown and overexpression experiments. They found mild but significant defects in proliferation upon siRNA knockdown in Colo320 (high N-BLR expression) cells but not in SW620 (low N-BLR expression) cells. Next, they profiled for markers of apoptosis following N-BLR knockdown, and found increased levels of cleaved PARP-1, increased activity of both Caspase 8/9 (initiators of apoptosis) and Caspase 3/7 (effectors of apoptosis), and decreased levels of XIAP, an inhibitor of Caspase 3/7. Upon observing these apoptotic phenotypes in multiple cell lines, they performed corollary experiments by overexpressing N-BLR, and observed mild but significant decreases in Caspase activity. These results support the role of N-BLR as a key regulator of apoptosis in CRC.
The authors further explored the role of N-BLR in CRC by performing invasion and migration assays, and saw significantly reduced or enhanced ability of stably silenced or activated N-BLR cells, respectively, to invade and migrate. To further understand the mechanism behind the N-BLR phenotype, Rigoutsos et al. performed microarray profiling in stably silenced HCT116 cells, and observed significant dysregulation of genes involved in EMT. Specifically, knockdown of N-BLR resulted in upregulation of E-cadherin (CDH1) and downregulation of vimentin (VIM) and ZEB1. ZEB1 is a transcriptional regulator that represses epithelial marker genes (e.g., CDH1) and activates mesenchymal genes (e.g., VIM), driving the EMT process. The authors hypothesized that N-BLR might promote the EMT phenotype via crosstalk with ZEB1. To explore this potential connection, they focused on the miR-200 family of miRNAs, given the cytoplasmic enrichment of N-BLR and the previously described role of these miRNAs in promoting EMT via repression of ZEB1.
They started by using the rna22 algorithm to predict putative miRNA targets within the miR-200 family, and found that miR-141-3p and miR-200c-3p were predicted to target N-BLR. While the authors did not demonstrate direct binding of N-BLR to these miRNAs using a CLIP-based approach, a potential binding interaction was indirectly supported by several experiments, including corollary expression analysis and luciferase reporter assays. Specifically, they observed increased or decreased levels of miR-141/200c-3p upon N-BLR knockdown or overexpression, respectively. N-BLR and miR-141/200c-3p expression levels were negatively correlated in tissue microarray samples, and low levels of miR-141/200c-3p predicted poor outcomes for CRC patients (similar to high levels of N-BLR). After demonstrating that N-BLR could relieve miR-200c-3p repression of XIAP and increase resistance to 5-FU-induced apoptosis, Rigoutsos and colleagues looked to reveal the specific motifs within N-BLR required for interaction with miR-141/200c-3p.
First, the authors returned to the original 20-nt pyknon motif (pyk90) they identified in N-BLR, and noticed that a segment of the predicted miR-200c-3p binding site overlapped with the 5’ region of the pyknon motif. To investigate further, they constructed several N-BLR deletion variants and transfected HT-29 cells, along with miR-141-3p/200c-3p mimics. Not surprisingly, they found that while WT N-BLR could effectively reduce levels of both miRNAs, deletion mutants of each predicted miRNA binding site could not. Interestingly, the pyk90 deletion mutant was unable to regulate miR-200c-3p, while it maintained the ability to reduce miR-141-3p expression, supporting the hypothesis that pyk90 likely functions as a crucial part of the miR-200c-3p binding site of N-BLR. Similarly, they observed that while overexpression of WT N-BLR resulted in increased levels of ZEB1, ZEB1 levels did not change upon overexpression of N-BLR mutants, suggesting that the upregulation of ZEB1 by N-BLR is mediated by N-BLR’s ability to sequester miR-141/200c-3p and act as a miRNA “sponge”. They demonstrated the functional consequences of this pyknon-mediated regulation through in vitro and in vivo assays, observing that deletion of the N-BLR pyk90 motif resulted in the loss of N-BLR-induced EMT phenotype in CRC lines.
To determine whether the functionality of the pyk90 region on EMT was due primarily to the region within the miR-200c-3p binding site, the authors constructed a second pyk90 mutant, deleting the region of pyk90 in-between the miR-200c-3p and miR-141-3p binding sites. Somewhat surprisingly, they observed no difference between the mutant and WT N-BLR in inducing migration or invasion of RKO and HCT116, suggesting that the only functionally relevant region of pyk90 resides in the 5-nt region overlapping the miR-200c-3p binding site. While Rigoutsos and colleagues state that this deletion mutant supports the critical role of the pyk90 motif in regulating N-BLR function, the evidence suggests that the majority of the motif is actually not required for miR-200c-3p sequestering, and the role of N-BLR in promoting EMT lies not in the pyknon region, but in the miR-141-3p and miR-200c-3p binding sites.
The authors end by performing genome-wide microarray profiling of pyknon transcripts in a variety of tissues. Interestingly, they observed that expression of pyknons appeared to be more tissue-specific than miRNAs and expressed at relatively high levels within samples. However, out of the thousands of pyknons examined, they only found a handful of transcripts associated with overall survival of CRC patients. Furthermore, they postulate that pyknons could serve as potential biomarkers, yet describe how a set of over 100 pyknon transcript probes could serve as an independent prognosis factor for overall survival. Despite the speculative nature of these claims, the tissue-specific nature and abundance of pyknons makes them promising regions for further investigation.
In summary, the study by Rigoutsos et al. adds N-BLR to the growing list of lncRNAs that act as functional ceRNAs within the context of cancer. Mechanistic studies are required to fully understand the function of N-BLR in mediating EMT, including CLIP experiments to verify miR-141/200c-3p binding and IP-MS of N-BLR to identify potential protein binding partners. A similar study (16) published in 2015 revealed that H19 promotes EMT in CRC by functioning as a miRNA sponge against members of the miR-200 family, supporting the idea that N-BLR likely acts within a network of ceRNAs that together regulate functional pathways within CRC. Indeed, studies revealing the dynamic roles of lncRNAs will help elucidate regulatory networks that may have significant therapeutic potential.
Acknowledgments
Funding: None.
Footnote
Provenance and Peer Review: This article was commissioned and reviewed by Section Editor Jinzhe Zhou (Department of General Surgery, Tongji Hospital, Tongji University School of Medicine, Shanghai, China).
Conflicts of Interest: Both authors have completed the ICMJE uniform disclosure form (available at http://dx.doi.org/10.21037/ncri.2017.10.03). The authors have no conflicts of interest to declare.
Ethical Statement: The authors are accountable for all aspects of the work in ensuring that questions related to the accuracy or integrity of any part of the work are appropriately investigated and resolved.
Open Access Statement: This is an Open Access article distributed in accordance with the Creative Commons Attribution-NonCommercial-NoDerivs 4.0 International License (CC BY-NC-ND 4.0), which permits the non-commercial replication and distribution of the article with the strict proviso that no changes or edits are made and the original work is properly cited (including links to both the formal publication through the relevant DOI and the license). See: https://creativecommons.org/licenses/by-nc-nd/4.0/.
References
- Ponting CP, Oliver PL, Reik W. Evolution and functions of long noncoding RNAs. Cell 2009;136:629-41. [Crossref] [PubMed]
- Ivey KN, Srivastava D. microRNAs as developmental regulators. Cold Spring Harb Perspect Biol 2015;7:a008144 [Crossref] [PubMed]
- Rinn JL, Chang HY. Genome regulation by long noncoding RNAs. Annu Rev Biochem 2012;81:145-66. [Crossref] [PubMed]
- Gifford CA, Ziller MJ, Gu H, et al. Transcriptional and epigenetic dynamics during specification of human embryonic stem cells. Cell 2013;153:1149-63. [Crossref] [PubMed]
- Liu SJ, Horlbeck MA, Cho SW, et al. CRISPRi-based genome-scale identification of functional long noncoding RNA loci in human cells. Science 2017;355:aah7111 [Crossref] [PubMed]
- Cabili MN, Trapnell C, Goff L, et al. Integrative annotation of human large intergenic noncoding RNAs reveals global properties and specific subclasses. Genes Dev 2011;25:1915-27. [Crossref] [PubMed]
- Lalevée S, Feil R. Long noncoding RNAs in human disease: emerging mechanisms and therapeutic strategies. Epigenomics 2015;7:877-9. [Crossref] [PubMed]
- Wapinski O, Chang HY. Long noncoding RNAs and human disease. Trends Cell Biol 2011;21:354-61. [Crossref] [PubMed]
- Wang J, Liu X, Wu H, et al. CREB up-regulates long non-coding RNA, HULC expression through interaction with microRNA-372 in liver cancer. Nucleic Acids Res 2010;38:5366-83. [Crossref] [PubMed]
- Li J, Zhang Q, Fan X, et al. The long noncoding RNA TUG1 acts as a competing endogenous RNA to regulate the Hedgehog pathway by targeting miR-132 in hepatocellular carcinoma. Oncotarget 2017;8:65932-45. [PubMed]
- Das S, Ghosal S, Sen R, et al. lnCeDB: database of human long noncoding RNA acting as competing endogenous RNA. PLoS One 2014;9:e98965 [Crossref] [PubMed]
- Li JH, Liu S, Zhou H, et al. starBase v2.0: decoding miRNA-ceRNA, miRNA-ncRNA and protein-RNA interaction networks from large-scale CLIP-Seq data. Nucleic Acids Res 2014;42:D92-7. [Crossref] [PubMed]
- Thomson DW, Dinger ME. Endogenous microRNA sponges: evidence and controversy. Nat Rev Genet 2016;17:272-83. [Crossref] [PubMed]
- Poliseno L, Salmena L, Zhang J, et al. A coding-independent function of gene and pseudogene mRNAs regulates tumour biology. Nature 2010;465:1033-8. [Crossref] [PubMed]
- Yan Y, Fan Q, Wang L, et al. LncRNA Snhg1, a non-degradable sponge for miR-338, promotes expression of proto-oncogene CST3 in primary esophageal cancer cells. Oncotarget 2017;8:35750-60. [PubMed]
- Liang WC, Fu WM, Wong CW, et al. The lncRNA H19 promotes epithelial to mesenchymal transition by functioning as miRNA sponges in colorectal cancer. Oncotarget 2015;6:22513-25. [Crossref] [PubMed]
- Rigoutsos I, Lee SK, Nam SY, et al. N-BLR, a primate-specific non-coding transcript leads to colorectal cancer invasion and migration. Genome Biol 2017;18:98. [Crossref] [PubMed]
- Ling H, Spizzo R, Atlasi Y, et al. CCAT2, a novel noncoding RNA mapping to 8q24, underlies metastatic progression and chromosomal instability in colon cancer. Genome Res 2013;23:1446-61. [Crossref] [PubMed]
- Rokavec M, Öner MG, Li H, et al. IL-6R/STAT3/miR-34a feedback loop promotes EMT-mediated colorectal cancer invasion and metastasis. J Clin Invest 2014;124:1853-67. [Crossref] [PubMed]
- Di Ruscio A, Ebralidze AK, Benoukraf T, et al. DNMT1-interacting RNAs block gene-specific DNA methylation. Nature 2013;503:371-6. [Crossref] [PubMed]
Cite this article as: Haswell JR, Slack FJ. Enabling EMT: N-BLR promotes metastasis in colorectal cancer via regulation of epithelial-to-mesenchymal transition. Non-coding RNA Investig 2017;1:14.