MicroRNAs in cell cycle progression and proliferation: molecular mechanisms and pathways
Introduction
The miRNA are small molecules of double stranded non-coding RNA of about 23 nucleotides and are present in animals, plants and viruses (1). Its main function is to regulate the gene expression of cellular receptors by the degradation of mRNA target molecules or by preventing their translation due to binding in the untranslated region (UTR) sequence (2). Encoded by genomic DNA, the miRNAs have their genes generally transcribed by RNA polymerase II in the cell nucleus (3).
Those molecules can be found intracellular or extracellular, including in various biological fluids such as blood, tears, urine, saliva, peritoneal fluid, cerebrospinal fluid or amniotic fluid and their expression is related to specific compartments (4-6). Specific miRNAs are released by their respective cells under certain stress conditions, either by active secretion or by cellular apoptosis (7).
The miRNAs are biomarkers of high specificity, selectivity and stability, and can be used in several areas, including clinical diagnostic analysis or forensic medicine (8). Extracellular miRNAs can also be found in exosomes, microvesicles and high-density lipoproteins, in which they have greater stability due to their formation of complexes with proteins that protect them from denaturation (9). Therefore, miRNAs play important regulatory roles in different imbalances and physiological processes (10).
Different miRNAs are expressed by cells of the immune system, possessing the ability to regulate innate and adaptive immune responses (3). In this context, miRNAs have become important biomarkers of signaling mechanisms that assist in the diagnosis and clinical management of some pathologies such as cardiovascular, metabolic, neurological diseases, neurological, infections, sepsis and different types of cancer (5,11,12).
Although miRNAs possess various known functions, data that prove their efficacy as biomarkers or therapeutic mechanisms are still conflicting since these molecules have a great variety in different populations studied (13). Therefore, we summarize the current findings of some miRNAs, such as miR-199b-5p, miR-193a-5p, miR-125b-5p, miR-125b-5p, miR-30a-5p, miR-27b-3p, which are involved in the regulation of proliferative and cell cycle pathways, as well as can used as biomarkers and cancer-anti therapy. Furthermore, we discuss the potential of these miRNAs in relation as proliferative and cell cycle regulators.
Cell cycle
Cell proliferation is a natural process that occurs in cells where a parent cell divides into two daughter cells (14). In eukaryotic cells, there are two distinct types of cell division: (I) meiosis, phase in which the parent cell divides producing two daughter cells with half of the genetic code of the mother cell, originating the gametes; and (II) in mitosis, the mother cells divide to give rise to two identical daughter cells (14). Whereas, the cell division cycle is divided into three phases: interphase, mitotic phase and cytokinesis (14,15). The interphase is the longest phase of the cell cycle, on average the cell spends about 90% of the time at this stage, obtaining nutrients, growing and doubling its DNA molecules, and thus preparing itself for the cellular division (14,15). The mitotic phase (M phase) is the nuclear division of the cells. At this stage, it begins with the condensation of the chromosomes, followed by their replication and division (14,15). In the last phase, cytokinesis, there is a rupture of the plasma membrane and completing the division process, giving rise to two daughter cells (14,15).
In addition, the interphase is divided into three sub-phases: G1, S and G2. In G1 phase, the cell has a high metabolic rate, producing a greater number of RNA and proteins, characterized by cellular growth (14,15). In S phase, the cell begins the process of multiplication of the genetic code resulting in the duplication of the chromatids. The G2 phase occurs after chromosome replication, with the polarization of the centrioles and the beginning of the mitotic spindle formation (14,15).
Upon completion of the G2 phase, begins the first phase of mitosis, prophase, in which the chromosomes begin to condense and initiates the synthesis of microtubules. (14,16). Then, prometaphase is initiated, where the nuclear membrane is degraded, and the microtubules reach the condensed chromosomes (14,16). In the metaphase, the chromosomes are aligned in the center of the cell, giving rise to the mitotic plate (14,16). The next phase, anaphase, the chromosome centromeres duplicate giving rise to two independent chromosomes that will migrate to opposite poles of the cell (14,16). In the last phase of mitosis, the telophase, the chromosomes are decondensed and a new nuclear membrane begins to be formed, and cell division is completed with the onset of cytokinesis and consequently formation of the daughter cells (14,16).
That process requires regulatory mechanisms, known as checkpoints that are related to its effectiveness. The division process is important for the normal physiology of tissues, since it can recover tissues and cells that have suffered damage or loss of function. Failures in these mechanisms are related to a series of pathological processes, such as cancer (17,18). To ensure the correct cellular replication, cell cycle have three major check points the G1 checkpoint, responsible for identifying DNA and cellular integrity; the G2/M checkpoint, identify DNA errors after replication; and the metaphase checkpoint, responsible to identify correct alignment of the chromosomes with the mitotic plate.
Cyclins and cyclin-dependent kinases (CDKs)
Cyclins (cyc) are proteins that control the progression of the cell cycle, their action is conditioned to the formation of a complex with CDKs (19,20). Cyclins can be divided into four classes, G1 cyclins, G1/S cyclins, S cyclins, and M cyclins. Each class of cyclin has the function of regulating different phases of the cell cycle, which gives rise to their names (19,20).
The major regulators of G1 phase are the CDK4/cycD and CDK2/cycE complexes that regulate DNA synthesis and G1 phase progression (21). These complexes can phosphorylate ribosomes by activating the expression of E2-factor (E2F) responsive genes, which encode proteins required for the G1/S phase transition, such as cycE, cycA, CDK, thymidine kinase (TK) and origin recognition complex subunit 1 (Orc1) (19). During G2 phase, the CDK2/cycA and CDK1/cycB complexes can phosphorylate the FoxM1 transcription factor, activating the necessary gene transcription to progress mitosis (19,22).
Growth factors (GF) and other mechanism of regulation
GF are usually proteins or steroid hormones that act as signaling molecules. Different GF can act on different cell types, through specific receptors in these cells, the growth factor receptors, such as vascular endothelial growth factor receptor (VEGFR), fibroblast growth factor receptor (FGFR), which can be found in vascular endothelial cells and fibroblasts, respectively (23,24). Those molecules are essential stimuli for the beginning of the process of cell division and differentiation.
These signals can act in the initial phase of G1 (23). The activation of growth factor receptors can trigger the activation of Ras, a G protein responsible for activating intracellular signaling pathways, which activate the mitogen-activated protein kinase (MAPK) signaling pathway (25). Another mechanism of signaling is through Wnt signaling pathway, which act through β-catenin promoting the activation of the myc transcription factor, responsible for increased transcription of cycD (26,27).
The tumor suppression factor, p53, is a transcription factor that activates the transcription of WAF1, an important G1/S phase-stop related protein, capable of forming complexes with CDK1, 2 and 4 inhibiting their activity (28). Other G1/S phase regulator is the retinoblastoma protein (pRb), this protein binds to the promoter domain of the E2F gene inhibiting the transcription of important proteins related to the progression of G1/S phase, such as cycA and cycE (29,30).
miRNAs in cellar proliferation
Several studies demonstrated the role of miRNAs as regulators of proliferation and cell cycle (31-34). However, the role of miRNA in these processes still needs to be better elucidated, since it may have opposite effects depending on its location. Here, we show the key role of some miRNAs (miR-199b-5p, miR-193a-5p, miR-125b-5p, miR-125b-5p, miR-30a-5p, miR-27b-3p) affecting different stages of cell cycle progression (Figure 1).
MiR-199b-5p role on cellular proliferation
The miR-199b-5p belonging to the miR-199b family member is located on chromosome 9 and is encoded in the reverse strand of the 2.2 kb intronic region between exons 14 and 15 of the dynamin 1 (DNM1) gene (31,32). This miRNA can act on both cancerous tissues and healthy tissues. In this regard, the miR-199b-5p may interfere with proliferation by regulation of specific de genes, such as human epidermal growth factor receptor-2 (HER2), laminin subunit gamma 1 (LAMC1), mixed lineage kinase-3 (MLK3), which are involved in the regulation of signaling pathways. Several studies also show that miR-199b-5p expression may be up or down regulated the proliferation process as shown below (35-37). The main mechanisms of miR-199b-5p on cellular cycle and proliferation are summarized on Figure 2.
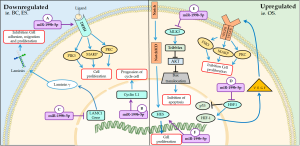
Downregulated
Some studies reported that patients who have poor prognosis in breast cancer express low levels of miR-199b-5p. MiR-199b-5p is responsible for inhibiting the expression of HER2, a transmembrane glycoprotein, which is associated with a phenotype more aggressive tumor (36,38). The receptor activation forms homodimers or heterodimers that autophosphorylate tyrosine residues followed by intracellular signaling pathways such as phosphatidylinositol-4,5-bisphosphate 3-kinase (PI3K), mitogen-activated MAPK and protein kinase C (PKC). Signaling such pathways results in the induction of proliferation, as well as cancer cell survival, motility and adhesion (39,40).
The downregulation of miR-199b-5p was also observed in cell lines Ewing’s sarcoma (ES) when compared to human mesenchymal stem cells (MSCs). Additional experiments suggest that expression of this miRNA acts as a suppressor of proliferation and arrest in the G1 to S cell cycle in sarcoma lines by inhibition of expression of cyclin L1 gene (CCNL1) (41). CCNL1 is a gene induced by GF, such as epidermal growth factor, which regulates cell cycle progression. This gene is involved in the processing of pre-mRNA for cell cycle transition G0 to G1 (42,43).
Yoo et al. identified that during cellular senescence the miR-199b-5p was notably downregulated. Cell senescence is a natural process of loss of the proliferative capacity of the cells through an irreversible stop of the cell cycle that occurs in the G0 phase. The results obtained by Yoo et al. further reveal that miR-199b-5p can suppress the translation activity of LAMC1, one of the subunit of the extra cellular matrix glycoprotein that indirectly regulates cellular senescence (35). This glycoprotein plays a role in the presentation of the basement membrane component by binding to the integrin that regulates signal transduction and acts to promote cell adhesion, migration and proliferation (44,45).
Upregulated
After the lipofection to up de miR-199b-5p, Du et al. observed that in mouse myocardial microvascular endothelial cells (MMVECs), there was a reduction in the expression of Heat shock transcription factor 1 (HSF1)/vascular endothelial growth factor (VEGF) blocking microvascular proliferation (46). HSF1 acts in the prevention of apoptosis and promotion of cardiac angiogenesis thus played a cardioprotective role. This protein can inhibit p53, cell cycle blocking protein, and increase hypoxia-inducing transcription factor-1 (HIF-1), thus promoting VEGF expression. VEGF acts on endothelial cells after dimerization and autophosphorylation, activating pathways such as PK C and PK D, PI3K and MAPK (47).
Sato-Kunisada et al. demonstrated that the up-regulation of miR-199b-5p in 60% partial pancreatectomy tissue was able to increase the proliferation of pancreatic β-Cells by upregulation of mixed lineage kinase-3 (MLK3) (37). This serine/threonine protein kinase acts as MAP3K to regulate K-RAS effector pathways by activating c-Jun amino-terminal kinase (JNK), extracellular signal regulated kinase (ERK) and p38 pathways. Studies performed on cancer cell lines demonstrate that MLK3 promotes cell proliferation (48,49). However, such a protein also implies cytokine signaling as well as cell death. In a study carried out by Pan et al. report that MLK3-JNK3 signaling is involved in neuronal apoptosis after ischemia/reperfusion injury (50). In addition, MLK3 has been reported to interact with Tribbles-3, a mammalian homolog of Drosophila Tribbles, whose function is to inhibit AKT activity by interacting with Akt pseudo-kinase inhibitor thereby suppressing cell survival in pancreatic cells (51).
Increased expression of miR-199b-5p was observed in osteosarcoma tissues of patients with poor prognosis (52). Won et al. also showed that this miRNA might influence the Notch signaling pathway on osteosarcoma cells, which performs cellular functions such as differentiation, survival and proliferation. Inhibition of this miRNA increased the expression of HES1, which is a transcription factor of the notch pathway, promoting a metastatic phenotype and promote cell growth (53,54)
MiR-193a-5p in cell proliferation
The miR-193a-5p belong to the miR-193a family and is located on human chromosome 17q11.2 (chr17:31,558,803-31,560,358). The miR-193a gene is transcribed into a precursor (pre-miR-193a) with 88 nucleotides, this pre-miR generates two mature miRNAs, miR-193a-3p and miR-193a-5p, depending on the arm that is processed during miRNA biogenesis (55). The miR-193a-5p is highly conserved across several species, such as homo sapiens, pan troglodytes, pongo pygmaeus and macaca mulatta besides canis familiaris and equus caballus, as indicated in the microRNA viewer database (56).
The effect of miR-193a-5p is still not well defined; according to literature, it has a dual effect depending on the regulation; and which gene is targeting. Fisher et al. reported that the miR-193a-5p act regulating genes of apoptosis, cell adhesion, regulation of epithelial-mesenchymal transition (EMT), blood vessel morphogenesis, cell cycle and of cytoskeleton rearrangement (57). In addition, others studies showed that this miR is downregulated suppressed proliferation and promoted apoptosis several tumors (57,58). However, Yang et al. demonstrated that mir-193a-5p is upregulated and promotes proliferation and tumor progression (59). The main mechanisms of miR-193b-5p on cellular cycle and proliferation are summarized on Figure 3.
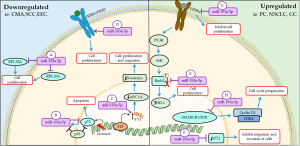
Downregulated
D’Argenio et al. investigated the functional consequences of miR-193a-5p downregulation in samples of blood of child with cow’s milk allergy and they identified that two genes were upregulated and are involved with proliferation (60). The first is 60S ribosomal protein L35a (RPL35A) gene, which encoding a protein called RPL35A, a 110-amino-acid ribosomal protein, is one structural component of the 60S ribosomal subunit and can be found in cytosol or extracellular region. This protein is required for the proliferation and viability of hematopoietic cells (61,62). Another gene involved is tumor protein p73 (TP73) gene that encodes p73. This protein along with p53 and p63, constitutes the p53 family, and play key roles in development, tumorigenesis and the response to DNA damage (63,64).
Previously, Ory and Ellisen proposed a miR-mediated negative feed-forward loop maintains p63/p73 homeostasis in the epithelium. In this study, these authors related in human that squamous cell carcinoma (SCC) p63 is a transcriptional repressor of miR-193a-5p and was activated by p73, besides TAp73 would be involved in negative feedback regulation of miR-193a and p63 inhibits p73 function. They confirm this theory with direct regulation of the p73 3'untranslated (3'UTR) by a transfected miR-193a mimic (65).
The p73 expression also is modulated by transcription E2F, the main target of pRb, plays essential roles in cell proliferation in the G1/S cell cycle checkpoint (66). Yin Yang 1 (YY1) induces transcriptional activity of p73 in synergism with E2F1 (67). Human YY1, consists of 414 amino acids, is a highly conserved transcription factor across species and ubiquitously expressed in human tissues (68,69). The YY1 is involved in cell cycle control and oncogenesis trough interaction with cycD, p53, c-Myc and pRb (67,70). According to Yang et al. in some cancer, for example endometrioid endometrial carcinoma (EEC) cells, YY1 protein is upregulated through downregulated miR-193a-5p expression and subsequently APC-1A gene is silenced through recruitment of EZH2 resulting in inactivation of APC, β-catenin activation and nuclear translocation to induce the expression of downstream target genes. The expression of these genes leads to increased cell proliferation and migration (67).
The miR-193a-5p can also directly regulated receptors such as ErbB2/HER2 by interacting with 3'UTR (71). The ErbB2 gene encodes a transmembrane glycoprotein and have an important role in cell growth, survival, and differentiation in normal cells (72). Patients with cancer that exhibit overexpression of ErbB2 is correlated with a poor clinical prognosis (33,73). According to Lin et al., the miR-193a-5p expression level was downregulated in samples of patients with esophageal squamous cell carcinoma (ESCC) while ERBB2/HEU2 was elevated promoting increase cell proliferation (33).
Upregulated
In several studies, miR-193a-5p was downregulated in many tumors, suggesting that it may be an important tumor inhibitor. On the other hand, in some type of cancer it is upregulated. Yang et al. showed that miR-193a-5p was upregulated in prostate cancer (PC) tissues and PC3 cell lines and promotes heme oxygenase-1 (HO-1) expression (59). HO-1 is a microsomal enzyme, 32 kDa protein that is constitutively expressed in the inner medullary zone of the kidneys, Kupffer cells, Purkinje cells in cerebellum and CD4+/CD25+ regulatory T lymphocytes. In addition, HO-1 is associated with response against oxidative stress and maintain cellular homeostasis (74).
According to Yang et al., demonstrated in vitro model, which after transfection of miR-193a-5p mimic into PC3 cells Bach2 expression was decreased (59). Under normal conditions, Bach2, a transcriptional repressor of the bZip TF family, is phosphorylated via PI3K/S6K and retained in the cytoplasm leading to repair and survival of the cell. However, under oxidative stress, Bach2 is dephosphorylated and translocated to the nucleus by disruption of nuclear-dependent Crm-1 resulting in apoptosis (75,76).
On the other hand, in breast cancer cells, the miR-193a overexpression inhibits the migration and invasion these cells by modulating Wilms’ tumor gene (WT1) expression (75). WT1 is a complex gene with 10 exons, located at chromosome 11p13, encodes a protein consisting of four zinc finger domains and can enhanced proliferation through upregulation of cycD1 and pRb (58,77).
Chen et al. also reported overexpression of miR-193a partially reversed tumor growth factor β1 (TGF-β1)- induced EMT in non-small cell lung cancers (NSCLC) cells (78). In this study, the miR reduced the also expression of WT1 that is negatively regulated the E-cadherin. Moreover, the miR-193a also act as a tumor suppressor by inhibited invasion by negatively regulating ERBB4/PIK3R3/mTOR/S6K2 and play a role in inhibiting proliferation and invasion and accelerating the apoptosis of lung cancer cells (79).
Upregulation of miR-193a-5p in childhood chordomas (CCs) is involved of TGF-β pathway and downregulation of SWI/SNF-related matrix-associated actin-dependent regulator of chromatin subfamily B member 1 (SMARCB1/INI1). SMARCB1 is one of the core subunit proteins in the SWItch/Sucrose Non-Fermentable (SWI/SNF) ATP-dependent chromatin remodeling complex encoded at chromosomal position 22q11.2 (80,81). Several studies have suggested that SMARCB1/INI1 suppresses tumor progression by signaling through the p16INK4a and pRb tumor suppressors to negatively to regulate cell cycle progression from G0/G1 to the S-phase. In cases of decreased of SMARCB1/INI1 leads to increased cycD1 which propagates the cell through the G1–S checkpoint (80).
MiR-125b-5p role on cellular proliferation
The miR-125 family is a human homologue of the first discovered microRNA, miR-lin-4, its members are involved in several cellular processes, such as cell differentiation, apoptosis, cell migration, immune response, pain signaling and cellular metabolism (82). Its expression can occur at the level of chromosome, gene or transcriptional unit (83). This family may act as tumor suppressor by inactivating a tumor suppressor gene or a gene related to a protein, which functions as a regular function of cell proliferation in different organs (84).
MiR-125b-5p, a member of this family, it has been reported as an important marker in the diagnosis of several diseases, such as acute myocardial infarction (AMI) (85), breast cancer (86), hepatocellular carcinoma (CHC) related to hepatitis B virus (HBV) (87), obstructive renal injury (88). In addition, it acts as a tumor suppressor in the proliferation of cancer cells under different transcription factors and is downregulated in tumor tissues (89). The main mechanisms of miR-125b-5p on cellular cycle and proliferation are summarized on Figure 4.
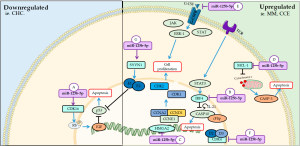
Downregulated
In vitro and in vivo studies showed that the down-regulation of miR-125b-5p in CHC leads to up-regulation of CDK16. Moreover, it plays an important role in tumor progression by increasing proliferation, facilitating cell cycle progression and inhibiting apoptosis via E2F1 (34). CDK16 regulates the cell cycle in cancer cells by interacting with p27KIP1 and catalyzing phosphorylation of the pRb, releasing E2F1, responsible for activating genes that participate in DNA synthesis (90). Expression of E2F1 transcription factor facilitates the up-regulation of cyclins in the cell cycle by binding DNA to specific proteins and this complex controls cell cycle progression at the G1/S phase transition (90,91). Some in vitro studies have shown that inhibition of CDK16 may be associated with various cancers (92).
Upregulated
On the other hand, up-regulation of miR-125b-5p inhibited the proliferation of multiple myeloma (MM) cells, according to in vivo and in vitro study, by acting as a negative regulator of interferon regulatory factor 4 (IRF4) (93). IRF4 is a specific factor of lymphocytes and when downregulated, it activates the casp-10/cFlip heterodimer leading to cell cycle arrest in G1 and cell apoptosis (94,95). In response to T cell receptor stimulation, activation of signal transducer and activator of transcription 3 (STAT3), a transcription factor that induces the expression of IRF4 to promote the differentiation of these cells mediated by IL-21 (96,97).
Overexpression of miR-125b-5p is able to inhibit cell proliferation and increase senescence of tumor cells by reducing high mobility group AT-hook 2 (HMGA2), a highly expressed transcription regulatory protein in esophageal carcinomas. In addition, this mRNAs also decreased the expression of the cell cycle regulatory genes CCNA2, CCND1 and CCNE1, cyclin coders that activate CDK1 and CDK2 and control the G1/S and G2/M transition phases (98).
In colon and rectum cancer cells, the overexpression of miR-125b-5p inhibited proliferation, leading to cell cycle blockade of the G2/M phase and inducing apoptosis via myeloid cell leukemia 1 (MCL1) and migration and invasion via granulocyte colony stimulating factor (G-CSF) (99). G-CSF is a hematopoietic glycoprotein that acts on G-CSF receptor (G-CSFR) to promote proliferation and differentiation of bone marrow cells and its migration to the blood through proteins such as JAK, STAT and Erk1 (100). This is glycoprotein induced this miRNA to suppress the proliferation of colon and rectum cancer cell lines, but promoted its migration and invasion, showing its metastatic effect in this pathology (99).
In normal cell lines, the miR-125b-5p was also able to inhibit cell proliferation in rat preadipocytes by decreasing the expression of cycD2, D3 and CDK4 and lead to cell cycle arrest in the G1/S transition phase, functioning as a potential therapeutic target in metabolic diseases and obesity (101). CycD2, D3, CDK4, among others play important roles in the progression of the cell cycle in G1 phase, as for example the activation of CDKs (102).
In synovial cells, up-regulation of miR-125b-5p favors the inhibition of cell proliferation and induction of apoptosis via downregulation of synoviolin 1 (SYVN1) and activation of p53, but an excess in this mechanism can lead to the development of osteoarthritis (103). In human synovial fluids, SYVN1 protects cells against stress, such as nitric oxide (NO) metabolites are capable of promoting apoptosis (103,104).
MiR-30a-5p role on cellular proliferation
The miR-30 family contain five members that are encoded by six human genes located on chromosomes 1, 6 and 8. Among them is miR-30a-5p, encoded by chromosome 6, which may exhibit opposite behaviors as tumor suppressor or oncogenic, depending on its upregulation or downregulation. Thus, it can act as a biomarker for tumor growth and metastasis, and may interfere in a beneficial or malignant way in some processes of cell proliferation (105). The main mechanisms of miR-30a-5p on cellular cycle and proliferation are summarized on Figure 5.
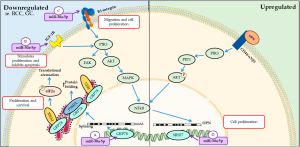
Downregulated
Studies showed that the downregulation of miR-30a-5p was able to inhibit the growth of renal cell carcinoma (RCC) through the downregulation of GRP78 expression, playing a suppressive role of the disease (106). According to Zhu and Lee, levels of GRP78 are directly related to the rate of cell proliferation and GRP78, which is a chaperone regulating endoplasmic reticulum (ER) homeostasis. These components are important in synthesis of secretory proteins of membranes and lipids, avoiding the accumulation of unfolded proteins, if it is overexpressed contributes for stress promoting cell proliferation (106,107). Under stress conditions, ER induces transcription of GRP78, which enhances protein folding in association with P58IPK and induces unfolded protein response (UPR) signaling in association with PERK and IRE1, thus contributing to cell survival and proliferation (106,108).
Downregulation of miR-30a-5p was also demonstrated in cases of gastric carcinoma (GC) related to the high expression of insulin-like growth factor receptor 1 (IGF-1R) (109). IGF-1R belongs to a family of tyrosine kinase receptors and is a facilitator of mitogenic synthesis. In addition, it may lead to the recruitment of substrates necessary for the activation of some intracellular pathways that regulate cell growth, such as PI3K/Akt and Ras/MAPK pathways mediated by FOXO and BAD, stimulating proliferation and inhibiting apoptosis (110,111).
By a downregulation of miR-30a-5p, the proliferation of malignant cells in mammary and rectal cervix tissue may be related to overexpression of lactate dehydrogenase (LDHA), ubiquitin protein ligase E3C (UBE3C) and β3 integrin (ITGβ3) (112-115). During estrogen-dependent mitotic cell, UBE3C interacts with estrogen receptors, the higher the expression of this protein, the greater can lead the interaction, with increased mitosis the cell will proliferate more (116). LDHA is an enzyme that catalysis the interconversion of pyruvate and NADH to lactate and NAD+ and plays a key role in regulating glycolysis, its overexpression generates more energy to the cell, increasing the frequency of cell metabolism as well as proliferation (117). ITGβ3 are membrane receptors responsible for several activities such as adhesion, migration and proliferation, activating a FAK/PI3K/AKT signaling cascade, which once overexpressed their functions also be increased (118).
Belonging to the serine family, fibroblast activation protein (FAP) is overexpressed due to downregulation of miR-30a-5p (119,120). Its expression is elevated in tissues under stress, which is able to increase the proliferation of tumor cells by capturing substrates into the cell through its exopeptidase activity by hydrolyzing N-terminal peptide bonds and endopeptidase by capturing glycine (121). Proliferation of cancer cells at high expression of profilin 2 (PFN-2) and beclin-1 are also related to (122). PFN-2 acts in the regulation of cellular iron, responsible for maintaining its homeostasis (118). In turn beclin-1 is related to the proliferation of drug-resistant cancer cells due to autophagy that this protein is able to trigger (122,123).
In another study, N-acetylgalactosaminyltransferase 7 (GALNT7) was related to the downregulation of miR-30a-5p, which proved to be a suppressor of RCC growth by modulating the expression of these genes. This fact suggests that negative regulation of this gene by miR-30a-5p could abolish alterations in malignant behavior (124). GALNT7 encodes and metabolizes cell surface, serum and extracellular matrix O-glycosides and when unregulated it distributes to the Golgi complex, reaching the ER, leading to uncontrolled cell proliferation (115,124).
Upregulated
According to Boguslawska et al. upregulation of miR-30a-5p was related to overexpression of SRSF7. The expression of this splicing factor regulates osteopontin (OPN), secreted in the extracellular matrix, being a positive indicator for some types of cancer, such as gastric, hepatic and renal, found in high plasma levels in these patients. In this regard, the suppression of SRSF7 is followed by a decrease in the proliferation of these cancer cells due to a change in OPN expression found in the extracellular matrix (125,126). OPN is a phosphorylated glycoprotein produced by different cell types, which interacts with the integrin CD44, and its variants and receptors, leading to the activation of cell survival pathways, angiogenesis, extracellular matrix degradation and also proliferation (126,127). In proliferation, when it binds to its receptors, OPN, follows the pathway PI3K/AKT/MAPK, inducing NF-κB that leads to cell proliferation (127).
MiR-27b-3p role on cellular proliferation
MiR-27b-3p is an intragenic miRNA located on chromosome 9q22.1 in the C9orf3 gene belonging to the miR-27b family. The regulation of miR-27b can occur mainly by genomic loss, epigenetic changes, transcriptional regulation, multiple molecules and signaling pathways (128).
Transcription factors and miRNA regulatory proteins play a critical role in the modulation of miR-27b-3p cell levels, both its downregulating and upregulating. These effects can lead the inhibition or activation of cellular functions, such as inhibition proliferation of tumor cells (e.g., breast cancer), and regulation of the cell cycle generally in the G1/G0 phase (129). The main mechanisms of miR-27b-3p on cellular cycle and proliferation are summarized on Figure 6.
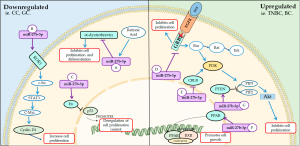
Downregulated
It is proven that miR-27b-3p downregulation is associated with exogenous oncogenes, such as the E6 gene from human papillomavirus (HPV) infection (129). Honegger et al. reported negative regulation of miR-27b-3p related to the inhibition of the E6 oncogene through transfection methods with synthetic siRNAs and cell cycle analyzes by assays with HPV18 positive tumor cells. This study defined the correlation of positive regulation of miR-27b-3p levels by p53, showing the ability of the protein encoded by the E6 gene to degrade the p53 cell cycle regulatory protein. The induction of degradation of p53 by the E6 oncoprotein results in a disturbed function of this important cell cycle protein after HPV infection (129,130).
The miR-27b-3p is well described as regulator in different types of cancer and in the case of gastric cancer. In this regard, Tao et al. found downregulation of this miR by overexpression in tumor cells of a transmembrane protein belonging to the receptor tyrosine kinase family, which is the orphan receptor 1 (ROR1) (131,132). In addition, the positive regulation of ROR1 is associated with phosphorylation and activation of c-Src (131). The ROR1-miR-27b-3p negative regulatory relationship promotes cancer progression, and the c-Src/STAT3 signaling pathway is involved in the regulation of cell proliferation (131). This fact suggests that the miR-27b-3p suppresses the proliferation, migration and invasion of gastric cancer cells and inhibits the growth and metastasis of gastric cancer (133).
Unlike a tumor context, miR-27b-3p is able to inhibit proliferation of non-tumor cells, for example in myoblasts. In addition, Li et al. showed that the retinoic acid (RA), required in organogenesis and myogeny, play a critical role in the regulation of miR-27b-3p (134). The alteration in the expression of RA-induced miR-27b-3p is involved with the regulation of α-dystrobrevin (DTNA). The DTNA is a cytoskeletal component that compose its structure and is important in signaling muscle cells, thereby participating in proliferation, gene regulation, and other processes, as well as DTNA 3'UTR is a direct target of miR-27b-3p (134).
Upregulated
Although not all regulatory mechanisms of miR-27b-3p are clearly elucidated to date, it is known that the upregulation of this microRNA is associated with an antiproliferative action (135). Liu et al. reported the ability of miR-27b-3p to reduce tumor growth in vitro, as well as the reduction of metastasis through its inhibition in triple-negative breast cancer (TNBC). This study also showed that miR-27b-3p is correlated with peroxisome proliferator-activated receptor (PPAR) transcription factor, which after activation, undergoes heterodimerization with retinoid X receptor (RXR), and phosphatase and tensin homolog (PTEN) signaling. Then, these complex regulates PI3K signaling by dephosphorylation of 3,4,5-triphosphate (PIP3), the main activator of the Akt cell survival kinase, negatively affecting the AKT/PI3K pathway, one of the most important pathways for cell growth, proliferation and survival (136-139).
The role of miR-27b-3p regulation in the synthesis of ovarian hormones, induction of granulosa cell proliferation and insulin resistance has also been defined through transcription factors not fully elucidated (140). Some studies have reported that miR-27b-3p may lead to activation of the PPAR pathway, such as Song et al. that showed that miR-27b might have PPARγ as the direct target, thereby having a vital role of this miR in porcine oocyte maturation and proliferation (141).
In addition, the functions of miR-27b can vary and even antagonistic, especially in proliferation, due this miR may have pro or anti-proliferative action, depending on the specific type of cancer. Chen et al. found that miR-27b levels decreased in breast cancer tissues, and their upregulation significantly inhibited the cell proliferation and promoted apoptosis through the downregulation of their target genes, such as CBLB and GRB2, which inactive the MAPK/Erk and PI3K/Akt signaling pathways (142).
Concluding remarks
Despite the growing interest in the study of miRNA, little is known about its involvement as regulator of physiological processes. The mentioned miRNAs appear to be involved in several processes of the cell cycle, as summarized in Table 1. Overall, these miRNAs can act on different proteins responsible for the regulation of cell cycle pathways, especially modulating the expression and activity of cyclins, CDKs, CKI and GF. Although its effects under regulatory proteins are well established, the same miRNA can regulate different proteins, showing a dual effect of induction or inhibition of cell proliferation or activating mechanisms of cell death, especially apoptosis. For example, the miR-193a-5p, which is related to three mechanisms, altering the expression patterns in different cell type, similar to other miRNAs described. In addition, a single miRNA may have different targets, showing a low specificity of the molecule in gene regulation, as well as miRNA expression may be different among distinct cell populations (144,145). These differences can be associated with distinct response of the same miRNA in these populations or with compensatory mechanism to preserve the cell function. On the other hand, some miRs regulate the expression of molecular targets in combination as miR-199b-5p and miR-30a-5p, thereby contributing to increased cell proliferation, metastasis, and inhibition of apoptosis of tumor cells. Thus, these molecules can be used to identify possible targets of these processes, as a potential biomarker for diagnosis and prognosis of several cancers. However, further studies are needed to improve knowledge about the mechanisms and functions of miRNAs in cell cycle progression and proliferation. Whereas, abnormalities in miRNA expression may be potent cancer development indicators, as well as changes in miRs and their molecular targets can also be important therapeutic strategy for these diseases.
Table 1
miR | Target genes | miR expression | Samples | Effect(s) | Ref. |
---|---|---|---|---|---|
miR-199a-5p | HER2↑ | Downregulated | Breast cancer tissues | Promotes cell proliferation and survival | (36,38) |
CCNL1↑ | Downregulated | Cell lines Ewing’s sarcoma | Promotes cell cycle | (41-43) | |
LAMC1↓ | Downregulated | Mesenchymal stem cells | Inhibit cell adhesion, proliferation, and migration | (35,143) | |
HSF1/VEGF↓ | Upregulated | Mouse myocardial microvascular endothelial cells | Inhibit cell proliferation and migration | (46) | |
MLK3↓ | Upregulated | Pancreas tissue post PPx | Inhibit apoptosis | (37,51) | |
Hes1↑ | Upregulated | Osteosarcoma tissues | Differentiation, survival, and proliferation | (52) | |
miR-193a-5p | RPL35A↑ | Downregulated | Blood of child with cow’s milk allergy | Promotes cell proliferation | (60) |
TAp73↑ | Downregulated | Blood of child with cow’s milk allergy | Apoptosis | (60,64) | |
YY1↑ | Downregulated | Endometrioid endometrial carcinoma cells | Promotes cell growth | (67) | |
ERBB2/HEU2↑ | Downregulated | Esophageal squamous cell carcinoma | Promotes cell proliferation | (33) | |
HO-1↑ Bach2↓ | Upregulated | Prostate cancer cells tissues and cell lines | Promotes cell proliferation | (59) | |
WT1↓ | Upregulated | Breast carcinoma tissues; non-small cell lung cancers (NSCLC) | Inhibit migration and invasion of cells | (58,75) | |
ERBB4/PIK3R3/mTOR/S6K2↓ | Upregulated | Lung cancer cells | Inhibits cell proliferation | (79) | |
SMARCB1/INI1↓ | Upregulated | Childhood chordomas | Cell cycle progression | (81) | |
miR-125b-5p | CDK16↑ via E2F1 | Downregulated | Hepatocellular carcinoma cells | Increase cell proliferation and inhibits apoptosis | (34) |
IRF4↓ | Upregulated | Multiple myeloma cells | Inhibits cell proliferation | (93) | |
HMGA2↓ | Overexpression | Esophageal carcinoma cells | Increase cell proliferation and senescence of tumor cells | (98) | |
G-CSF↓ and MCL1↓ | Overexpression | Cancer cells of the colon and rectum | Inhibits cell proliferation and induces apoptosis | (99) | |
cyclins D2, D3 and CDK4↓ | Upregulated | Pre-adipocytes | Inhibits cell proliferation | (101) | |
SYVN1↓ | Upregulated | Synovial cells | Inhibits cell proliferation and induces apoptosis | (103) | |
miR-30a-5p | GRP78↓; GALNT-7↑; | Downregulated | Human cancer renal tissue and cell line of mouse | Inhibit cell proliferation | (106,107,113,125) |
LDHA↑; UBE3C↑; Integrin β3↑ | Downregulated | Human mammary cancerous tissue | Increase cell proliferation, migration and adhesion | (118,122,123,126) | |
IGF-1R↑ | Downregulated | Human gastric cancerous tissue | Increase cell proliferation and inhibits apoptosis | (110,112,127) | |
FAP↑ | Downregulated | Human cancerous oral tissue | Increase cell proliferation | (109) | |
PFN-2↑; BECLIN-1↑ | Downregulated | Cells of the human pulmonary epithelium | Increase cell proliferation and autophagy | (108,111) | |
SRSF7↑ | Upregulated | Mice cell line | Increase cell proliferation | (120) | |
miR-27b-3p | E6/E7↑ | Downregulated | HPV18 positive tumor cells | Deregulation of cell proliferation control | (129) |
ROR1↑ | Downregulated | Human gastric cancer cells | Increase cell proliferation | (131) | |
DTNA↑ | Downregulated | Myoblast | Inhibits cell proliferation and differentiation | (134) | |
Steroidogenesis and folliculogenesis genes↓ | Upregulated | Granulosa cells | Promotes cell growth | (135) | |
PPAR/PTEN↓ | Upregulated | Triple-negative breast cancer cells | Inhibits cell proliferation | (136) |
PPx, partial pancreatectomy.
Acknowledgments
Funding: The authors were supported by the Brazilian’s agencies: Conselho Nacional de Desenvolvimento Científico e Tecnológico (CNPq), Coordenação de Aperfeiçoamento de Pessoal de Nível Superior (CAPES), Fundação Amazônia Paraense de Amparo à Pesquisa (FAPESPA), Federal University of Pará and MC Monteiro thanks for the fellowship from CNPq.
Footnote
Conflicts of Interest: All authors have completed the ICMJE uniform disclosure form (available at http://dx.doi.org/10.21037/ncri.2018.04.06). MCM serves as an unpaid editorial board member of Non-coding RNA Investigation from June 2017 to May 2019. The other authors have no conflicts of interest to declare.
Ethical Statement: The authors are accountable for all aspects of the work in ensuring that questions related to the accuracy or integrity of any part of the work are appropriately investigated and resolved.
Open Access Statement: This is an Open Access article distributed in accordance with the Creative Commons Attribution-NonCommercial-NoDerivs 4.0 International License (CC BY-NC-ND 4.0), which permits the non-commercial replication and distribution of the article with the strict proviso that no changes or edits are made and the original work is properly cited (including links to both the formal publication through the relevant DOI and the license). See: https://creativecommons.org/licenses/by-nc-nd/4.0/.
References
- Bernardo BC, Ooi JY, Lin RC, et al. miRNA therapeutics: a new class of drugs with potential therapeutic applications in the heart. Future Med Chem 2015;7:1771-92. [Crossref] [PubMed]
- Balusu S, Van Wonterghem E, De Rycke R, et al. Identification of a novel mechanism of blood–brain communication during peripheral inflammation via choroid plexus‐derived extracellular vesicles. EMBO Mol Med 2016;8:1162-83. [Crossref] [PubMed]
- O’Connell RM, Rao DS, Chaudhuri AA, et al. Physiological and pathological roles for microRNAs in the immune system. Nat Rev Immunol 2010;10:111-22. [Crossref] [PubMed]
- Mittelbrunn M, Gutiérrez-Vázquez C, Villarroya-Beltri C, et al. Unidirectional transfer of microRNA-loaded exosomes from T cells to antigen-presenting cells. Nat Commun 2011;2:282. [Crossref] [PubMed]
- Reithmair M, Buschmann D, Märte M, et al. Cellular and extracellular miRNAs are blood-compartment-specific diagnostic targets in sepsis. J Cell Mol Med 2017;21:2403-11. [Crossref] [PubMed]
- Nagarkatti M, Rieder SA, Hegde VL. Do cannabinoids have a therapeutic role in transplantation? Trends Pharmacol Sci 2010;31:345-50. [PubMed]
- Dumache R, Rogobete AF, Bedreag OH, et al. Use of miRNAs as biomarkers in sepsis. Anal Cell Pathol (Amst) 2015;2015:186716
- Roderburg C, Luedde M, Vargas Cardenas D, et al. Circulating microRNA-150 serum levels predict survival in patients with critical illness and sepsis. PLoS One 2013;8:e54612 [Crossref] [PubMed]
- Rabinowits G, Gerçel-Taylor C, Day JM, et al. Exosomal microRNA: a diagnostic marker for lung cancer. Clin Lung Cancer 2009;10:42-6. [Crossref] [PubMed]
- Qin Y, Wu L, Ouyang Y, et al. MiR-125a Is a critical modulator for neutrophil development. PLoS Genet 2017;13:e1007027 [Crossref] [PubMed]
- Monteiro VV, Reis JF, de Souza Gomes R, et al. Dual behavior of exosomes in septic cardiomyopathy. In: Xiao J, Cretoiu S. editors. Exosomes in Cardiovascular Diseases. Advances in Experimental Medicine and Biology, vol 998. Springer, Singapore, 2017:101-12.
- Bei Y, Das S, Rodosthenous RS, et al. Extracellular Vesicles in Cardiovascular Theranostics. Theranostics 2017;7:4168-82. [Crossref] [PubMed]
- Wang J, Chen J, Sen S. MicroRNA as Biomarkers and Diagnostics. J Cell Physiol 2016;231:25-30. [Crossref] [PubMed]
- Rhind N, Russell P. Signaling pathways that regulate cell division. Cold Spring Harb Perspect Biol 2012;4:a005942 [Crossref] [PubMed]
- Duronio RJ, Xiong Y. Signaling pathways that control cell proliferation. Cold Spring Harb Perspect Biol 2013;5:a008904 [Crossref] [PubMed]
- Wieser S, Pines J. The biochemistry of mitosis. Cold Spring Harb Perspect Biol 2015;7:a015776 [Crossref] [PubMed]
- Paduch R. Theories of cancer origin. Eur J Cancer Prev 2015;24:57-67. [Crossref] [PubMed]
- Rycaj K, Tang DG. Cell-of-origin of cancer versus cancer stem cells: Assays and interpretations. Cancer Res 2015;75:4003-11. [Crossref] [PubMed]
- Bendris N, Lemmers B, Blanchard JM. Cell cycle, cytoskeleton dynamics and beyond: the many functions of cyclins and CDK inhibitors. Cell Cycle 2015;14:1786-98. [Crossref] [PubMed]
- Lim S, Kaldis P. Cdks, cyclins and CKIs: roles beyond cell cycle regulation. Development 2013;140:3079-93. [Crossref] [PubMed]
- Bertoli C, Skotheim JM, De Bruin RA. Control of cell cycle transcription during G1 and S phases. Nat Rev Mol Cell Biol 2013;14:518-28. [Crossref] [PubMed]
- Gire V, Dulic V. Senescence from G2 arrest, revisited. Cell Cycle 2015;14:297-304. [Crossref] [PubMed]
- Witsch E, Sela M, Yarden Y. Roles for Growth Factors in Cancer Progression. Physiology (Bethesda) 2010;25:85-101. [Crossref] [PubMed]
- Sweeney C, Fambrough D, Huard C, et al. Growth Factor-specific Signaling Pathway Stimulation and Gene Expression Mediated by ErbB Receptors. J Biol Chem 2001;276:22685-98. [Crossref] [PubMed]
- Nussinov R, Tsai CJ, Jang H. Oncogenic ras isoforms signaling specificity at the membrane. Cancer Res 2018;78:593-602. [Crossref] [PubMed]
- Stewart DJ. Wnt signaling pathway in non-small cell lung cancer. J Natl Cancer Inst 2014;106:djt356 [Crossref] [PubMed]
- Sharma G, Sharma AR, Seo EM, et al. Genetic polymorphism in extracellular regulators of Wnt signaling pathway. Biomed Res Int 2015;2015:847529
- Speidel D. The role of DNA damage responses in p53 biology. Arch Toxicol 2015;89:501-17. [Crossref] [PubMed]
- Uchida C. The Retinoblastoma Protein: Functions Beyond the G1-S Regulator. Curr Drug Targets 2012;13:1622-32. [Crossref] [PubMed]
- Giacinti C, Giordano A. RB and cell cycle progression. Oncogene 2006;25:5220-7. [Crossref] [PubMed]
- Favreau AJ, McGlauflin RE, Duarte CW, et al. miR-199b, a novel tumor suppressor miRNA in acute myeloid leukemia with prognostic implications. Exp Hematol Oncol 2016;5:4. [Crossref] [PubMed]
- Li Y, Bai H, Zhang Z, et al. The Up-Regulation of miR-199b-5p in Erythroid Differentiation Is Associated with GATA-1 and NF-E2. Mol Cells 2014;37:213-9. [Crossref] [PubMed]
- Lin CH, Tsai CH, Yeh CT, et al. MiR-193a-5p/ERBB2 act as concurrent chemoradiation therapy response indicator of esophageal squamous cell carcinoma. Oncotarget 2016;7:39680-93. [PubMed]
- Wang Y, Qin X, Guo T, et al. Up-regulation of CDK16 by multiple mechanisms in hepatocellular carcinoma promotes tumor progression. J Exp Clin Cancer Res 2017;36:97. [Crossref] [PubMed]
- Yoo JK, Kim CH, Jung HY, et al. Discovery and characterization of miRNA during cellular senescence in bone marrow-derived human mesenchymal stem cells. Exp Gerontol 2014;58:139-45. [Crossref] [PubMed]
- Fang C, Zhao Y, Guo B. MiR-199b-5p targets HER2 in breast cancer cells. J Cell Biochem 2013;114:1457-63. [Crossref] [PubMed]
- Sato-Kunisada R, Yoshida N, Nakamura S, et al. Enhanced Expression of miR-199b-5p Promotes Proliferation of Pancreatic beta-Cells by Down-Regulation of MLK3. Microrna 2016;5:57-65. [Crossref] [PubMed]
- Fang C, Wang FB, Li Y, et al. Down-regulation of miR-199b-5p is correlated with poor prognosis for breast cancer patients. Biomed Pharmacother 2016;84:1189-93. [Crossref] [PubMed]
- Krishnamurti U, Silverman JF. HER2 in breast cancer: A review and update. Adv Anat Pathol 2014;21:100-7. [Crossref] [PubMed]
- Iqbal N, Iqbal N. Human Epidermal Growth Factor Receptor 2 (HER2) in Cancers: Overexpression and Therapeutic Implications. Mol Biol Int 2014;2014:852748
- Li W, Li Y, Guo J, et al. Overexpression of miR-199b-5p inhibits Ewing’s sarcoma cell lines by targeting CCNL1. Mol Med Rep 2015;12:3359-64. [Crossref] [PubMed]
- Sticht C, Hofele C, Flechtenmacher C, et al. Amplification of Cyclin L1 is associated with lymph node metastases in head and neck squamous cell carcinoma (HNSCC). Br J Cancer 2005;92:770-4. [Crossref] [PubMed]
- Muller D, Millon R, Théobald S, et al. Cyclin L1 (CCNL1) gene alterations in human head and neck squamous cell carcinoma. Br J Cancer 2006;94:1041-4. [Crossref] [PubMed]
- Aumailley M. The laminin family. Cell Adh Migr 2013;7:48-55. [Crossref] [PubMed]
- Ning L, Kurihara H, De Vega S, et al. Laminin α1 regulates age-related mesangial cell proliferation and mesangial matrix accumulation through the TGF-β pathway. Am J Pathol 2014;184:1683-94. [Crossref] [PubMed]
- Du P, Dai F, Chang Y, et al. Role of miR-199b-5p in regulating angiogenesis in mouse myocardial microvascular endothelial cells through HSF1/VEGF pathway. Environ Toxicol Pharmacol 2016;47:142-8. [Crossref] [PubMed]
- Wang S, Li X, Parra M, et al. Control of endothelial cell proliferation and migration by VEGF signaling to histone deacetylase 7. Proc Natl Acad Sci U S A 2008;105:7738-43. [Crossref] [PubMed]
- Zhang J, Lu L, Xiong Y, et al. MLK3 promotes melanoma proliferation and invasion and is a target of microRNA-125b. Clin Exp Dermatol 2014;39:376-84. [Crossref] [PubMed]
- Chen J, Miller EM, Gallo KA. MLK3 is critical for breast cancer cell migration and promotes a malignant phenotype in mammary epithelial cells. Oncogene 2010;29:4399-411. [Crossref] [PubMed]
- Pan J, Zhang QG, Zhang GY. The neuroprotective effects of K252a through inhibiting MLK3/MKK7/JNK3 signaling pathway on ischemic brain injury in rat hippocampal CA1 region. Neuroscience 2005;131:147-59. [Crossref] [PubMed]
- Humphrey RK, Newcomb CJ, Yu SMA, et al. Mixed lineage kinase-3 stabilizes and functionally cooperates with TRIBBLES-3 to compromise mitochondrial integrity in cytokine-induced death of pancreatic beta cells. J Biol Chem 2010;285:22426-36. [Crossref] [PubMed]
- Zeng H, Zhang Z, Dai X, et al. Increased Expression of microRNA-199b-5p Associates with Poor Prognosis Through Promoting Cell Proliferation, Invasion and Migration Abilities of Human Osteosarcoma. Pathol Oncol Res 2016;22:253-60. [Crossref] [PubMed]
- Gao F, Huang W, Zhang Y, et al. Hes1 promotes cell proliferation and migration by activating Bmi-1 and PTEN/Akt/GSK3β pathway in human colon cancer. Oncotarget 2015;6:38667-80. [Crossref] [PubMed]
- Won KY, Kim YW, Kim HS, et al. MicroRNA-199b-5p is involved in the Notch signaling pathway in osteosarcoma. Hum Pathol 2013;44:1648-55. [Crossref] [PubMed]
- Grossi I, Salvi A, Abeni E, et al. Biological Function of MicroRNA193a-3p in Health and Disease. Int J Genomics 2017;2017:5913195
- Kiezun A, Artzi S, Modai S, et al. MiRviewer: A multispecies microRNA homologous viewer. BMC Res Notes 2012;5:92. [Crossref] [PubMed]
- Fisher JN, Terao M, Fratelli M, et al. MicroRNA networks regulated by all-trans retinoic acid and Lapatinib control the growth, survival and motility of breast cancer cells. Oncotarget 2015;6:13176-200. [Crossref] [PubMed]
- Chen J, Gao S, Wang C, et al. Pathologically decreased expression of MIR-193a contributes to metastasis by targeting WT1-E-cadherin axis in non-small cell lung cancers. J Exp Clin Cancer Res 2016;35:173. Erratum in: J Exp Clin Cancer Res 2017;36:31. [Crossref] [PubMed]
- Yang Z, Chen JS, Wen JK, et al. Silencing of miR-193a-5p increases the chemosensitivity of prostate cancer cells to docetaxel. J Exp Clin Cancer Res 2017;36:178. [Crossref] [PubMed]
- D’Argenio V, Del Monaco V, Paparo L, et al. Altered miR-193a-5p expression in children with cow’s milk allergy. Allergy 2018;73:379-86. [Crossref] [PubMed]
- Farrar JE, Dahl N. Untangling the phenotypic heterogeneity of diamond Blackfan anemia. Semin Hematol 2011;48:124-35. [Crossref] [PubMed]
- Nakao A, Yoshihama M, Kenmochi N. RPG: the Ribosomal Protein Gene database. Nucleic Acids Res 2004;32:D168-70. [Crossref] [PubMed]
- Yoon MK, Ha JH, Lee MS, et al. Structure and apoptotic function of p73. BMB Rep 2015;48:81-90. [Crossref] [PubMed]
- Ory B, Ramsey MR, Wilson C, et al. A microRNA-dependent program controls p53-independent survival and chemosensitivity in human and murine squamous cell carcinoma. J Clin Invest 2011;121:809-20. [Crossref] [PubMed]
- Ory B, Ellisen LW. A microRNA-dependent circuit controlling p63/p73 homeostasis: p53 family cross-talk meets therapeutic opportunity. Oncotarget 2011;2:259-64. [Crossref] [PubMed]
- Ankers JM, Awais R, Jones NA, et al. Dynamic NF-κb and E2F interactions control the priority and timing of inflammatory signalling and cell proliferation. Elife 2016;5: [Crossref] [PubMed]
- Yang Y, Zhou L, Lu L, et al. A novel miR-193a-5p-YY1-APC regulatory axis in human endometrioid endometrial adenocarcinoma. Oncogene 2013;32:3432-42. [Crossref] [PubMed]
- Ibarra L, Webb C. Advantages of Fuzzy Control While Dealing with Complex/ Unknown Model Dynamics: A Quadcopter Example, New Applications of Artificial Intelligence Pedro Ponce, IntechOpen. 2016. Available online: http://www.intechopen.com/books/new-applications-of-artificial-intelligence/advantages-of-fuzzy-control-while-dealing-with-complex-unknown-model-dynamics-a-quadcopter-example
- Khachigian LM. The Yin and Yang of YY1 in tumor growth and suppression. Int J Cancer 2018; [Epub ahead of print]. [Crossref] [PubMed]
- Zaravinos A, Spandidos DA. Yin yang 1 expression in human tumors. Cell Cycle 2010;9:512-22. [Crossref] [PubMed]
- Leivonen SK, Sahlberg KK, Mäkelä R, et al. High-throughput screens identify microRNAs essential for HER2 positive breast cancer cell growth. Mol Oncol 2014;8:93-104. [Crossref] [PubMed]
- Tan M, Yu D. Molecular mechanisms of ErbB2-mediated breast cancer chemoresistance. Adv Exp Med Biol 2007;608:119-29. [Crossref] [PubMed]
- Muthuswamy SK, Li D, Lelievre S, et al. ErbB2, but not ErbB1, reinitiates proliferation and induces luminal repopulation in epithelial acini. Nat Cell Biol 2001;3:785-92. [Crossref] [PubMed]
- Yadav B, Greish K. Selective inhibition of hemeoxygenase-1 as a novel therapeutic target for anticancer treatment. J Nanomedic Nanotechnol 2011;S4:005.
- Xie F, Hosany S, Zhong S, et al. MicroRNA-193a inhibits breast cancer proliferation and metastasis by downregulating WT1. PLoS One 2017;12:e0185565 [Crossref] [PubMed]
- Yoshida C, Yoshida F, Sears DE, et al. Bcr-Abl signaling through the PI-3 / S6 kinase pathway inhibits nuclear translocation of the transcription factor Bach2, which represses the antiapoptotic factor heme oxygenase-1. Blood 2007;109:1211-9. [Crossref] [PubMed]
- Sugiyama H. WT1 (Wilms’ tumor gene 1): Biology and cancer immunotherapy. Jpn J Clin Oncol 2010;40:377-87. [Crossref] [PubMed]
- Chen Q, Tong C, Ma S, et al. Involvement of microRNAs in probiotics-induced reduction of the cecal inflammation by Salmonella typhimurium. Front Immunol 2017;8:704. [Crossref] [PubMed]
- Liang H, Liu M, Yan X, et al. MiR-193a-3p functions as a tumor suppressor in lung cancer by down-regulating ERBB4. J Biol Chem 2015;290:926-40. [Crossref] [PubMed]
- Kohashi K, Oda Y. Oncogenic roles of SMARCB1/INI1 and its deficient tumors. Cancer Sci 2017;108:547-52. [Crossref] [PubMed]
- Malgulwar PB, Pathak P, Singh M, et al. Downregulation of SMARCB1/INI1 expres-sion in pediatric chordomas correlates with upregulation of miR-671-5p and miR-193a-5p expressions. Brain Tumor Pathol 2017;34:155-9. [Crossref] [PubMed]
- Yin H, Sun Y, Wang X, et al. Progress on the relationship between miR-125 family and tumorigenesis. Exp Cell Res 2015;339:252-60. [Crossref] [PubMed]
- Ha M, Kim VN. Regulation of microRNA biogenesis. Nat Rev Mol Cell Biol 2014;15:509-24. [Crossref] [PubMed]
- Esquela-Kerscher A, Slack FJ. Oncomirs - MicroRNAs with a role in cancer. Nat Rev Cancer 2006;6:259-69. [Crossref] [PubMed]
- Jia K, Shi P, Han X, et al. Diagnostic value of miR-30d-5p and miR-125b-5p in acute myocardial infarction. Mol Med Rep 2016;14:184-94. [Crossref] [PubMed]
- Matamala N, Vargas MT, González-Cámpora R, et al. Tumor MicroRNA expression profiling identifies circulating MicroRNAs for early breast cancer detection. Clin Chem 2015;61:1098-106. [Crossref] [PubMed]
- Giray BG, Emekdas G, Tezcan S, et al. Profiles of serum microRNAs; miR-125b-5p and miR223-3p serve as novel biomarkers for HBV-positive hepatocellular carcinoma. Mol Biol Rep 2014;41:4513-9. [Crossref] [PubMed]
- Wang S, Wu L, Du L, et al. Reduction in miRNA-125b-5p levels is associated with obstructive renal injury. Biomed Rep 2017;6:449-54. [Crossref] [PubMed]
- Yang D, Zhan M, Chen T, et al. MIR-125b-5p enhances chemotherapy sensitivity to cisplatin by down-regulating Bcl2 in gallbladder cancer. Sci Rep 2017;7:43109. [Crossref] [PubMed]
- Sheldon LA. Inhibition of E2F1 activity and cell cycle progression by arsenic via retinoblastoma protein. Cell Cycle 2017;16:2058-72. [Crossref] [PubMed]
- Kent LN, Bae S, Tsai SY, et al. Dosage-dependent copy number gains in E2f1 and E2f3 drive hepatocellular carcinoma. J Clin Invest 2017;127:830-42. [Crossref] [PubMed]
- Phadke M, Remsing Rix LL, Smalley I, et al. Dabrafenib inhibits the growth of BRAF-WT cancers through CDK16 and NEK9 inhibition. Mol Oncol 2018;12:74-88. [Crossref] [PubMed]
- Morelli E, Leone E, Cantafio ME, et al. Selective targeting of IRF4 by synthetic microRNA-125b-5p mimics induces anti-multiple myeloma activity in vitro and in vivo. Leukemia 2015;29:2173-83. [Crossref] [PubMed]
- Shaffer AL, Emre NC, Lamy L, et al. IRF4 addiction in multiple myeloma. Nature 2008;454:226-31. [Crossref] [PubMed]
- Lamy L, Ngo VN, Emre NC, et al. Control of autophagic cell death by caspase-10 in multiple myeloma. Cancer Cell 2013;23:435-49. [Crossref] [PubMed]
- Knudsen NH, Lee CH. IL-21 and IRF4: A Complex partnership in immune and metabolic regulation. Diabetes 2014;63:1838-40. [Crossref] [PubMed]
- Huber M, Brustle A, Reinhard K, et al. IRF4 is essential for IL-21-mediated induction, amplification, and stabilization of the Th17 phenotype. Proc Natl Acad Sci U S A 2008;105:20846-51. [Crossref] [PubMed]
- Mei LL, Wang WJ, Qiu YT, et al. miR-125b-5p functions as a tumor suppressor gene partially by regulating HMGA2 in esophageal squamous cell carcinoma. PLoS One 2017;12:e0185636 [Crossref] [PubMed]
- Zhang X, Ma X, An H, et al. Upregulation of microRNA-125b by G-CSF promotes metastasis in colorectal cancer. Oncotarget 2017;8:50642-54. [PubMed]
- Welte K G-CSF. filgrastim, lenograstim and biosimilars. Expert Opin Biol Ther 2014;14:983-93. [Crossref] [PubMed]
- Ouyang D, Ye Y, Guo D, et al. MicroRNA-125b-5p inhibits proliferation and promotes adipogenic differentiation in 3T3-L1 preadipocytes. Acta Biochim Biophys Sin (Shanghai) 2015;47:355-61. [Crossref] [PubMed]
- Hunter T, Pines J. Cyclins and Cancer .2. Cyclin-D and Cdk Inhibitors Come of Age. Cell 1994;79:573-82. [Crossref] [PubMed]
- Ge FX, Li H, Yin X. Upregulation of microRNA-125b-5p is involved in the pathogenesis of osteoarthritis by downregulating SYVN1. Oncol Rep 2017;37:2490-6. [Crossref] [PubMed]
- Farrell AJ, Blake DR, Palmer RM, et al. Increased concentrations of nitrite in synovial fluid and serum samples suggest increased nitric oxide synthesis in rheumatic diseases. Ann Rheum Dis 1992;51:1219-22. [Crossref] [PubMed]
- Yang SJ, Yang SY, Wang DD, et al. The miR-30 family: Versatile players in breast cancer. Tumour Biol 2017;39:1010428317692204 [Crossref] [PubMed]
- Wang C, Cai L, Liu J, et al. MicroRNA-30a-5p Inhibits the Growth of Renal Cell Carcinoma by Modulating GRP78 Expression. Cell Physiol Biochem 2017;43:2405-19. [Crossref] [PubMed]
- Zhu G, Lee AS. Role of the unfolded protein response, GRP78 and GRP94 in organ homeostasis. J Cell Physiol 2015;230:1413-20. [Crossref] [PubMed]
- Ni M, Zhang Y, Lee AS. Beyond the endoplasmic reticulum: atypical GRP78 in cell viability, signalling and therapeutic targeting. Biochem J 2011;434:181-8. [Crossref] [PubMed]
- Wang N, Wang L, Yang Y, et al. A serum exosomal microRNA panel as a potential biomarker test for gastric cancer. Biochem Biophys Res Commun 2017;493:1322-8. [Crossref] [PubMed]
- Renoir JM, Marsaud V, Lazennec G. Estrogen receptor signaling as a target for novel breast cancer therapeutics. Biochem Pharmacol 2013;85:449-65. [Crossref] [PubMed]
- Liu Y, Zhou Y, Gong X, et al. MicroRNA-30a-5p inhibits the proliferation and in-vasion of gastric cancer cells by targeting insulin-like growth factor 1 receptor. Exp Ther Med 2017;14:173-80. [Crossref] [PubMed]
- Wei W, Yang Y, Cai J, et al. MiR-30a-5p Suppresses Tumor Metastasis of Human Colorectal Cancer by Targeting ITGB3. Cell Physiol Biochem 2016;39:1165-76. [Crossref] [PubMed]
- Xiong J, Wei B, Ye Q, et al. MiR-30a-5p/UBE3C axis regulates breast cancer cell proliferation and migration. Biochem Biophys Res Commun 2016; [Epub ahead of print]. [Crossref] [PubMed]
- Li W, Liu C, Zhao C, et al. Downregulation of β3 integrin by miR-30a-5p modulates cell adhesion and invasion by interrupting Erk/Ets-1 network in triple-negative breast cancer. Int J Oncol 2016;48:1155-64. [Crossref] [PubMed]
- Hussain MR, Hoessli DC, Fang M. N-acetylgalactosaminyltransferases in cancer. Oncotarget 2016;7:54067-81. [Crossref] [PubMed]
- Okada M, Ohtake F, Nishikawa H, et al. Liganded ERα Stimulates the E3 Ubiquitin Ligase Activity of UBE3C to Facilitate Cell Proliferation. Mol Endocrinol 2015;29:1646-57. [Crossref] [PubMed]
- Jin L, Chun J, Pan C, et al. Phosphorylation-mediated activation of LDHA promotes cancer cell invasion and tumour metastasis. Oncogene 2017;36:3797-806. [Crossref] [PubMed]
- Zhang N, Ma D, Wang L, et al. Insufficient radiofrequency ablation treated hepatocellular carcinoma cells promote metastasis by up-regulation ITGB3. J Cancer 2017;8:3742-54. [Crossref] [PubMed]
- Ruan P, Tao Z, Tan A. Low expression of miR-30a-5p induced the proliferation and invasion of oral cancer via promoting the expression of FAP. Biosci Rep 2018;38:BSR20171027 [Crossref] [PubMed]
- Tian ZQ, Li ZH, Wen SW, et al. Identification of Commonly Dysregulated Genes in Non-small-cell Lung Cancer by Integrated Analysis of Microarray Data and qRT-PCR Validation. Lung 2015;193:583-92. [Crossref] [PubMed]
- Juillerat-Jeanneret L, Tafelmeyer P, Golshayan D. Fibroblast activation protein-α in fibrogenic disorders and cancer: more than a prolyl-specific peptidase? Expert Opin Ther Targets 2017;21:977-91. [Crossref] [PubMed]
- Yang X, Bai F, Xu Y, et al. Intensified Beclin-1 Mediated by Low Expression of Mir-30a-5p Promotes Chemoresistance in Human Small Cell Lung Cancer. Cell Physiol Biochem 2017;43:1126-39. [Crossref] [PubMed]
- Galluzzi L, Pietrocola F, Bravo-San Pedro JM, et al. Autophagy in malignant transformation and cancer progression. EMBO J 2015;34:856-80. [Crossref] [PubMed]
- Baker MA, Davis SJ, Liu P, et al. Tissue-Specific MicroRNA Expression Patterns in Four Types of Kidney Disease. J Am Soc Nephrol 2017;28:2985-92. [Crossref] [PubMed]
- Boguslawska J, Sokol E, Rybicka B, et al. microRNAs target SRSF7 splicing factor to modulate the expression of osteopontin splice variants in renal cancer cells. Gene 2016;595:142-9. [Crossref] [PubMed]
- Maeda N, Maenaka K. The roles of matricellular proteins in oncogenic virus-induced cancers and their potential utilities as therapeutic targets. Int J Mol Sci 2017;18: [Crossref] [PubMed]
- Cao DX, Li ZJ, Jiang XO, et al. Osteopontin as potential biomarker and therapeutic target in gastric and liver cancers. World J Gastroenterol 2012;18:3923-30. [Crossref] [PubMed]
- Ding L, Ni J, Yang F, et al. Promising therapeutic role of miR-27b in tumor. Tumour Biol 2017;39:1010428317691657 [Crossref] [PubMed]
- Honegger A, Schilling D, Bastian S, et al. Dependence of Intracellular and Exosomal microRNAs on Viral E6/E7 Oncogene Expression in HPV-positive Tumor Cells. PLoS Pathog 2015;11:e1004712 [Crossref] [PubMed]
- Stiasny A, Freier CP, Kuhn C, et al. The involvement of E6, p53, p16, MDM2 and Gal-3 inthe clinical outcome of patients with cervical cancer. Oncol Lett 2017;14:4467-76. [Crossref] [PubMed]
- Tao J, Zhi X, Zhang X, et al. MiR-27b-3p suppresses cell proliferation through targeting receptor tyrosine kinase like orphan receptor 1 in gastric cancer. J Exp Clin Cancer Res 2015;34:139. [Crossref] [PubMed]
- Zhang XY, Zhang PY. Receptor tyrosine kinases in carcinogenesis Oncol Lett 2016;12:3679-82. (Review). [Crossref] [PubMed]
- Feng Q, Wu X, Li F, et al. miR-27b inhibits gastric cancer metastasis by targeting NR2F2. Protein Cell 2017;8:114-22. [Crossref] [PubMed]
- Li N, Tang Y, Liu B, et al. Retinoid acid-induced microRNA-27b-3p impairs C2C12 myoblast proliferation and differentiation by suppressing α-dystrobrevin. Exp Cell Res 2017;350:301-11. [Crossref] [PubMed]
- Liu Y, Cai Q, Bao PP, et al. Tumor tissue microRNA expression in association with triple-negative breast cancer outcomes. Breast Cancer Res Treat 2015;152:183-91. [Crossref] [PubMed]
- Tachibana K, Yamasaki D, Ishimoto K, et al. The role of PPARs in cancer. PPAR Res 2008;2008:102737
- Berger J, Moller DE. The Mechanisms of Action of PPARs. Annu Rev Med 2002;53:409-35. [Crossref] [PubMed]
- Chu EC, Tarnawski AS. PTEN regulatory functions in tumor suppression and cell biology. Med Sci Monit 2004;10:RA235-41. [PubMed]
- Rowe DL, Ozbay T, O’Regan RM, et al. Modulation of the BRCA1 protein and induction of apoptosis in triple negative breast cancer cell lines by the polyphenolic compound curcumin. Breast Cancer (Auckl) 2009;3:61-75. [Crossref] [PubMed]
- Xie S, Batnasan E, Zhang Q, et al. MicroRNA Expression is Altered in Granulosa Cells of Ovarian Hyperresponders. Reprod Sci 2016;23:1001-10. [Crossref] [PubMed]
- Song C, Yao J, Cao C, et al. PPARγ is regulated by miR-27b-3p negatively and plays an important role in porcine oocyte maturation. Biochem Biophys Res Commun 2016;479:224-30. [Crossref] [PubMed]
- Chen D, Si W, Shen J, et al. MiR-27b-3p inhibits proliferation and potentially reverses multi-chemoresistance by targeting CBLB/GRB2 in breast cancer cells. Cell Death Dis 2018;9:188. [Crossref] [PubMed]
- Ke HL, Ke RH, Li B, et al. Association between laminin γ1 expression and meningioma grade, recurrence, and progression-free survival. Acta Neurochir (Wien) 2013;155:165-71. [Crossref] [PubMed]
- Rawlings-Goss RA, Campbell MC, Tishkoff SA. Global population-specific variation in miRNA associated with cancer risk and clinical biomarkers. BMC Med Genomics 2014;7:53. [Crossref] [PubMed]
- Huang RS, Gamazon ER, Ziliak D, et al. Population differences in microRNA expression and biological implications. RNA Biol 2011;8:692-701. [Crossref] [PubMed]
Cite this article as: Rodrigues DVS, Monteiro VVS, Navegantes-Lima KC, Oliveira ALDB, Gaspar SLDF, Quadros LBG, Monteiro MC. MicroRNAs in cell cycle progression and proliferation: molecular mechanisms and pathways. Non-coding RNA Investig 2018;2:28.