A novel p53-mediated miR-30a/ZEB2 regulatory network in the control of breast cancer progression
Understanding of the complexity of the microRNA (miRNA)-mediated regulatory network is still largely unknown for many diseases, including cancer. Recent evidences on the control of miRNA-mediated circuits in complex networks have contributed to address the intricate interplay through which miRNAs achieve their regulatory role (1). This editorial provides a summarizing view of a novel p53-mediated regulatory role of miR-30a/ZEB2 circuit that has been validated in the context of triple negative breast cancers (TNBC). A canonical regulatory network includes transcription factors, miRNAs and target genes (2). The article of di Gennaro et al. offers an example of an experimentally p53-regulated miRNA circuit involved in TNBC. TP53 (tumor protein p53) is the most frequently mutated gene in human cancers. It encodes a tetrameric transcription factor that in wild-type (wt; not mutant) conformation exerts tumor suppressor activities (3,4). Wt-p53 protein binds to response elements on regulatory regions of its target genes, and transcriptionally induces the production of proteins that reduce cell proliferation, trigger apoptosis and orchestrate DNA damage response (4). Besides transcribing coding genes, p53 also induces the transcription of miRNAs that participate in the p53 network contributing to the control on tumor progression (5).
p53-deficient cancers are often unstable, aggressive, and resistant to therapy, indicating a strong selective pressure for TP53 inactivation during tumor progression (3). p53 results mutated in approximately 80% of TNBC, a subgroup of basal-like breast cancers that lack both the estrogen and progesterone receptors and HER2. This subtype of breast cancers, that is actually poorly cured, is characterized by TP53 alterations, which are largely disrupting mutations (loss of tumor suppressive functions) (6-9). The loss of function of wt-p53 can confer a selective advantage to TNBC progression controlling the transcription of miRNAs on a variety of processes, including epithelial-mesenchymal transition (EMT) and cell plasticity (5-10). Therefore, a deep analysis of miRNA-mediated mechanisms through which p53 inactivation contributes to the invasive behavior of BC is of paramount importance to understand the interplay of known molecules in functional regulatory circuits.
In their study di Gennaro et al. contributed to highlight the p53/miRNA crosstalk in TNBC progression, by demonstrating the role of a new p53/miR-30a/ZEB2 axis. Starting from an in silico prediction, by employing The Cancer Genome Atlas (TCGA) dataset (9), the authors focused only on miR-30a, being the only miRNA downregulated in TP53-mutated tumors. miR-30a belongs to the miR-30 family that consists of five highly conserved miRNAs (miR-30a-e), which lie in various chromosomes or neighboring sites (11). miR-30a exerts different functions by regulating processes involved in tumor progression. In particular, miR-30a controls EMT by targeting Snai1 and Slug expression (12-14). In this regards, in high-grade-serous ovarian cancers, which represent the majority of OC characterized by 90% of TP53 mutations, miR-30a is associated with poor prognosis and chemoresistance (15). In ovarian cancer cells miR-30a directly targets the endothelin A receptor (ETAR) and, consequently, inhibits the ETAR-mediated increase of mesenchymal markers (Snail and vimentin) and E-cadherin downregulation. These events lead to reduced survival, cell migration, invasion, plasticity and platinum-response. These findings provide evidence of a hitherto unappreciated miR-30a function as a tumor suppressor in chemoresistant OC cells and its contribution to poor patient outcome. Accordingly, by downregulating vimentin, miR-30a inhibits breast cancer invasiveness and metastasis and reduced miR-30a levels are associated with a poor clinical outcome (16). In line with these evidences, di Gennaro et al. demonstrated that low miR-30a levels were associated with a poor clinical outcome in TNBC patients, thus suggesting a prognostic relevance of miR-30a in this BC subtype. Of note, a downregulation of both miR-30a strands (miR-30-3p/-5p) was observed in TP53-mutated BC, particularly TNBC, strongly supporting the existence of an in vivo p53/miR-30a interplay. Notably, re-expression of p53 in p53-deficient BC cell lines up-regulated both miR-30a strand expressions, acting on their primary transcript (pri-miR-30a). Accordingly with previous findings in melanoma cells (17), di Gennaro et al. reported that wt-p53 protein induced miR-30a transcription in TNBC by specifically binding its promoter in two binding regions (Figure 1). Among the direct targets of miR-30a the authors identified ZEB2, an E-box-binding transcription factor that is implicated in the regulation of E-cadherin expression (18). Of clinical relevance, ZEB2 is up-regulated in TNBC and basal-like BCs in which it associates with an aggressive tumor phenotype (19). With the identification of miR-30a as a p53-downstream effector in TNBC, the authors contributed to put in evidence the mechanistic link between p53 and ZEB2 in the control of tumor progression. Importantly, the authors also demonstrated the existence of a complex regulatory network that integrated miR-200c. This miRNA belongs to the miR-200 family that includes five members (miR-200a/b/c, miR-141 and miR-429) (20). Of interest, p53 inhibits EMT also by modulating the miR-200 expression and in TP53-mutated BC cells miR-200c is downregulated, supporting the notion that inactivation of p53 promotes EMT and thereby tumor progression and poor drug response (10,21,22). Moreover, miR-200 family and ZEB transcription factors reciprocally inhibit their expression through a negative feedback loop (23). In this work, the authors demonstrated that re-expression of miR-30a in TNBC cells led to ZEB2 downregulation and to the consequent miR-200c upregulation. Importantly, they found a positive association between miR-30a and miR-200c in TNBC patients, thus supporting the interplay of miR-200c with p53/miR-30a axis. Regarding the biological implications of this novel identified network in TNBC biology, they found that miR-30a/ZEB2 network controlled cell migration and invasiveness.
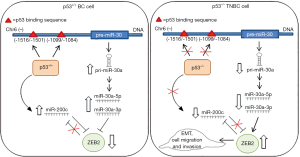
Collectively, di Gennaro et al. through the identification of the p53/miR-30a/ZEB2 circuit, integrated with miR-200c, contribute to reveal the molecular mechanisms by which loss of p53 induces EMT and aggressive features of BC disclosing how miR-30a achieves its regulatory role acting as a central node in this circuit (Figure 1). Summing up, this study highlights the role of p53 in regulating the functional properties of miRNA-mediated circuits, characterizing its pathological significance in TNBC context. Further studies are needed to validate the involvement of this network in the control of BC progression as well as its prognostic potential. To this end, it will be useful to analyze a larger cohort of BC patients with full clinical annotation available. To validate the prognostic relevance of this network, it could be important to assess the presence of the reported miRNA profile in the plasma along with the status of TP53 gene in BC patients at diagnosis and relapse. The analysis of circulating miRNA could also allow evaluating potential changes in the network miRNA/gene at diagnosis and relapse in BC patients. Most importantly, this analysis could be predictive of the impact of first-line treatment in BC. Genome wide approaches (e.g., Nanostring analysis) will be extremely important to quantify the expression and the alterations of this network in BC patients. Last, but not least, to fully understand the mechanisms underlying the function of the miRNA-regulatory network, the development of suitable mathematical models (24,25) might improve the interpretation of the complexity of these regulatory networks and thereby provide precious information elucidating the composition and function of these circuits in cancer progression.
Acknowledgments
Funding: This work was supported by Associazione Italiana Ricerca sul Cancro (AIRC) [IG 14199 to A Bagnato, IG 20613 to G Blandino].
Footnote
Provenance and Peer Review: This article was commissioned and reviewed by the Section Editor Dr. Rongrong Gao (Department of Cardiology, the First Affiliated Hospital of Nanjing Medical University, Nanjing, China).
Conflicts of Interest: All authors have completed the ICMJE uniform disclosure form (available at http://dx.doi.org/10.21037/ncri.2018.06.11). The authors have no conflicts of interest to declare.
Ethical Statement: The authors are accountable for all aspects of the work in ensuring that questions related to the accuracy or integrity of any part of the work are appropriately investigated and resolved.
Open Access Statement: This is an Open Access article distributed in accordance with the Creative Commons Attribution-NonCommercial-NoDerivs 4.0 International License (CC BY-NC-ND 4.0), which permits the non-commercial replication and distribution of the article with the strict proviso that no changes or edits are made and the original work is properly cited (including links to both the formal publication through the relevant DOI and the license). See: https://creativecommons.org/licenses/by-nc-nd/4.0/.
References
- Cora' D, Re A, Caselle M, Bussolino F. MicroRNA-mediated regulatory circuits: outlook and perspectives. Phys Biol 2017;14:045001 [Crossref] [PubMed]
- Nazarov PV, Reinsbach SE, Muller A, et al. Interplay of microRNAs, transcription factors and target genes: linking dynamic expression changes to function. Nucleic Acids Res 2013;41:2817-31. [Crossref] [PubMed]
- Bykov VJN, Eriksson SE, Bianchi J, et al. Targeting mutant p53 for efficient cancer therapy. Nat Rev Cancer 2018;18:89-102. [Crossref] [PubMed]
- Kastenhuber ER, Lowe SW. Putting p53 in context. Cell 2017;170:1062-78. [Crossref] [PubMed]
- Goeman F, Strano S, Blandino G. MicroRNAs as key effectors in the p53 network. Int Rev Cell Mol Biol 2017;333:51-90. [Crossref] [PubMed]
- Yadav BS, Chanana P, Jhamb S. Biomarkers in triple negative breast cancer: a review. World J Clin Oncol 2015;6:252-63. [Crossref] [PubMed]
- Kalimutho M, Parsons K, Mittal D, et al. Targeted therapies for triple-negative breast cancer: combating a stubborn disease. Trends Pharmacol Sci 2015;36:822-46. [Crossref] [PubMed]
- Perou CM, Sørlie T, Eisen MB, et al. Molecular portraits of human breast tumours. Nature 2000;406:747-52. [Crossref] [PubMed]
- Cancer Genome Atlas Network. Comprehensive molecular portraits of human breast tumors. Nature 2012;490:61-70. [Crossref] [PubMed]
- Chang CJ, Chao CH, Xia W, et al. p53 regulates epithelial-mesenchymal transition and stem cell properties through modulating miRNAs. Nat Cell Biol 2011;13:317-23. [Crossref] [PubMed]
- Yang X, Chen Y, Chen L. The versatile role of microRNA-30a in human cancer. Cell Physiol Biochem 2017;41:1616-32. [Crossref] [PubMed]
- Kumarswamy R, Mudduluru G, Ceppi P, et al. MicroRNA-30a inhibits epithelial-to-mesenchymal transition by targeting Snai1 and is downregulated in non-small cell lung cancer. Int J Cancer 2012;130:2044-53. [Crossref] [PubMed]
- Zhou Q, Yang M, Lan H. at al. miR-30a negatively regulates TGF-β1-induced epithelial-mesenchymal transition and peritoneal fibrosis by targeting Snai1. Am J Pathol 2013;183:808-19. [Crossref] [PubMed]
- Chang CW, Yu JC, Hsieh YH, et al. MicroRNA-30a increases tight junction protein expression to suppress the epithelial-mesenchymal transition and metastasis by targeting Slug in breast cancer. Oncotarget 2016;7:16462-78. [PubMed]
- Sestito R, Cianfrocca R, Rosanò L, et al. miR-30a inhibits endothelin A receptor and chemoresistance in ovarian carcinoma. Oncotarget 2016;7:4009-23. [Crossref] [PubMed]
- Cheng CW, Wang HW, Chang CW, et al. MicroRNA-30a inhibits cell migration and invasion by downregulating vimentin expression and is a potential prognostic marker in breast cancer. Breast Cancer Res Treat 2012;134:1081-93. [Crossref] [PubMed]
- Park D, Kim H, Kim Y, et al. miR-30a regulates the expression of CAGE and p53 and regulates the response to anti-cancer drugs. Mol Cells 2016;39:299-309. [Crossref] [PubMed]
- Gheldof A, Hulpiau P, van Roy F, et al. Evolutionary functional analysis and molecular regulation of the ZEB transcription factors. Cell Mol Life Sci 2012;69:2527-41. [Crossref] [PubMed]
- Karihtala P, Auvinen P, Kauppila S, et al. Vimentin, Zeb1 and Sip1 are up-regulated in triple-negative and basal-like breast cancers: association with an aggressive tumour phenotype. Breast Cancer Res Treat 2013;138:81-90. [Crossref] [PubMed]
- Humphries B, Yang C. The microRNA-200 family: small molecules with novel roles in cancer development, progression and therapy. Oncotarget 2015;6:6472-98. [Crossref] [PubMed]
- Kim T, Veronese A, Pichiorri F, et al. p53 regulates epithelial-mesenchymal transition through microRNAs targeting ZEB1 and ZEB2. J Exp Med 2011;208:875-83. [Crossref] [PubMed]
- Alam F, Mezhal F, El Hasasna H, et al. The role of p53-microRNA 200-moesin axis in invasion and drug resistance of breast cancer cells. Tumour Biol 2017;39:1010428317714634 [Crossref] [PubMed]
- Brabletz S, Brabletz T. The ZEB/miR-200 feedback loop--a motor of cellular plasticity in development and cancer? EMBO Rep 2010;11:670-7. [Crossref] [PubMed]
- Lai X, Wolkenhauer O, Vera J. Understanding microRNA-mediated gene regulatory networks through mathematical modelling. Nucleic Acids Res 2016;44:6019-35. [Crossref] [PubMed]
- Sorrells TR, Johnson AD. Making sense of transcription networks. Cell 2015;161:714-23. [Crossref] [PubMed]
Cite this article as: Sestito R, Blandino G, Bagnato A. A novel p53-mediated miR-30a/ZEB2 regulatory network in the control of breast cancer progression. Non-coding RNA Investig 2018;2:40.