Circulating microRNAs as modifiable diagnostic biomarkers of gestational and transgenerational metabolic risk: can exercise play a role?
Gestational diabetes mellitus (GDM) is defined as any degree of glucose intolerance at first recognition during pregnancy (1). It is usually diagnosed at 24–28 gestational weeks, according to the recommendations of international associations and committees (2). Diagnosis is made using a sequential model of universal screening with a 50-g one-hour glucose challenge test (GCT), followed by a diagnostic 100-g three-hour oral glucose tolerance test (OGTT) for women with a positive screening test (3). The overall incidence of GDM is increasing worldwide, affecting approximately 7–15% of all pregnancies (4). It is considered the most frequent metabolic problem during pregnancy, representing about 90% of risky pregnancies (5), although the prevalence of pregnancies complicated by diabetes (gestational or pre-gestational) varies geographically and among different ethnic groups (6). During healthy pregnancy insulin resistance increases due to the secretion of a series of placental hormones antagonists to insulin (cortisol, prolactin, progesterone and lactogen), ultimately causing blood glucose to rise (6). Furthermore, in GDM women insulin production is insufficient to counteract this increasing insulin resistance, due to an increase in β-pancreatic cells apoptotic rate which leads to an abnormal production and secretion of insulin (7). Given that blood glucose easily goes through placenta by facilitated diffusion, it reaches the fetus producing fetal hyperglycemia (8).
GDM has been associated with substantial adverse health outcomes for both mothers, who have considerably elevated risk for impaired type 2 diabetes mellitus (T2D) in the years following pregnancy, and the offspring, who are more likely to develop macrosomia, prenatal morbidity, prematurity and cardiomyopathy, as well as an increased risk of obesity and insulin resistance in adulthood (9), as a result of developmental programming (10).
The underlying pathogenic mechanisms behind this abnormal metabolic risk profile in the offspring are unknown, but epigenetic changes induced by this altered intra-uterine milieu are implicated (10). Among the epigenetic processes potentially involved, there is now increasing evidence that non-coding RNAs, like microRNAs (miRNAs), play a role in the development of metabolic diseases. In this sense, in recent years it has been shown that miRNAs regulate genes involved in metabolic processes such as glucose homeostasis, insulin signalling, pancreatic β-cell function, lipid metabolism, and inflammation (11,12).
miRNAs are short (18–25 nucleotides), noncoding RNA molecules that regulate posttranscriptional gene expression by promoting mRNA degradation or by repressing protein translation (13). miRNAs are not exclusively intracellular but they can be released from cells where they are generated and exist stably as circulating miRNAs (c-miRNAs) in blood and in other easily accessible biologic fluids (such as urine, saliva, amniotic fluid and breast milk), associated with proteins, extracellular vesicles (exosomes or microvesicles) or in a complex with lipoproteins (14,15). c-miRNAs can be actively or passively released from tissues and regulate the gene expression of distant cells, acting as a true intercellular communication mechanism in an autocrine, paracrine, or endocrine mode (16,17). Furthermore, Li et al. (18) demonstrated that small non-coding RNAs can be transplacentally transmitted from the mother to the fetus, contributing to the maternal-fetal crosstalk and to the epigenetic legacy.
In the last couple of years, a considerable amount of information has been published describing the association of GDM with the expression of certain miRNAs (6,19-26) (Figure 1). There are some evidences that dysregulation of miRNAs in GDM may arise from altered miRNA machinery. In this sense, Rahimi et al. (27) detected the upregulation of Drosha, Dicer and DGCR8 in blood samples of GDM women compared to healthy pregnant women. These results suggest that miRNAs are involved in the pathogenesis of GDM. Most studies have analyzed maternal blood samples (6,20), plasma or serum, during pregnancy, since it is an easily accessible and non-invasive fluid. In other studies, maternal samples were obtained after delivery, such as placental tissues (6,19,21,22,25,26), omental adipose tissue (6) and human umbilical vein endothelial cells (HUVECs) (6,23). Moreover, two studies have assessed miRNA expression in the offspring: one in skeletal muscle of adult offspring of women who had developed GDM (6) and the other in feto-placental endothelial cells (fpEC) (24).
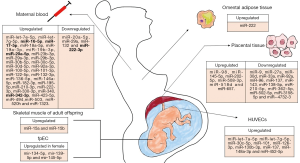
It has been observed that changes in the levels of certain c-miRNAs occur before changes in blood glucose become detectable, which underscores their potential value as early biomarkers of GDM. However, the information available about the usefulness and viability of c-miRNAs either as early biomarkers of GDM, to control the progression of the disease, or for therapeutic purposes (14) is scarce and, to a certain extent, contradictory and incomplete. In this sense, lifestyle variables could have a confounding effect, due to their influence on c-miRNA profiles.
It has been previously described that lifestyle factors, such as physical exercise, alter c-miRNA profile (17). Most of these studies demonstrate that acute exercise and training elicit changes in the type of c-miRNAs and its expression levels in both healthy and diseased people, including type-2 diabetic patients. This profile has been suggested to depend on the mode, intensity, and dose of exercise (17). Physical exercise is a useful tool to reduce the risk of GDM and it is a metabolic risk modifier for the mother and the fetus. In the management of women with GDM, there is evidence that exercise, structured aerobic and/or resistance training, is a beneficial adjunctive therapy (28). Moreover, maternal exercise performed before, during and after pregnancy has also been shown to provide significant benefits to the fetus health: increased amniotic fluid, placenta viability and volume, and vascular function; faster placenta growth and greater villous tissue; lower risk of macrosomia and preterm birth; improved neurodevelopment; and lower fetal body fat percentage (28). All these findings suggest that one of the mechanisms involved in this protection mediated by physical exercise could be miRNA expression and secretion to the blood stream.
Therefore, there is a need for understanding the effect of exercise performed during pregnancy, particularly by women developing GDM, on plasma c-miRNA profiles. This may help in explaining the role of these epigenetic regulators in the molecular pathways involved in the benefits exerted by regular exercise during gestation for diabetic pregnant women and in the reduction of metabolic risk to the offspring. Furthermore, combining both the c-miRNA signature and lifestyle variables, particularly exercise, will greatly enhance the accuracy of the predictive value of c-miRNAs as early biomarkers of GDM risk and may also permit trajectories of risk to be tracked to monitor the efficacy of interventions in order to avoid or decrease some metabolic risks for both mother and fetus (29).
Acknowledgments
Funding: P Pinto-Hernández acknowledges a financial aid for students of PhD Programs from University of Oviedo (2019-04801). E Iglesias-Gutiérrez acknowledges Grant PAPI-19-PUENTE-16 from University of Oviedo.
Footnote
Provenance and Peer Review: This article was commissioned by the editorial office, Non-coding RNA Investigation. The article did not undergo external peer review.
Conflicts of Interest: All authors have completed the ICMJE uniform disclosure form (available at http://dx.doi.org/10.21037/ncri.2019.08.01). The authors have no conflicts of interest to declare.
Ethical Statement: The authors are accountable for all aspects of the work in ensuring that questions related to the accuracy or integrity of any part of the work are appropriately investigated and resolved.
Open Access Statement: This is an Open Access article distributed in accordance with the Creative Commons Attribution-NonCommercial-NoDerivs 4.0 International License (CC BY-NC-ND 4.0), which permits the non-commercial replication and distribution of the article with the strict proviso that no changes or edits are made and the original work is properly cited (including links to both the formal publication through the relevant DOI and the license). See: https://creativecommons.org/licenses/by-nc-nd/4.0/.
References
- Bascones-Martinez A, Matesanz-Perez P, Escribano-Bermejo M, et al. Periodontal disease and diabetes-Review of the Literature. Med Oral Patol Oral Cir Bucal 2011;16:e722-9. [Crossref] [PubMed]
- Liu B, Xu Y, Zhang Y, et al. Early Diagnosis of Gestational Diabetes Mellitus (EDoGDM) study: a protocol for a prospective, longitudinal cohort study. BMJ Open 2016;6:e012315 [Crossref] [PubMed]
- Zhao C, Dong J, Jiang T, et al. Early second-trimester serum miRNA profiling predicts gestational diabetes mellitus. PLoS One 2011;6:e23925 [Crossref] [PubMed]
- Ferrara A. Increasing prevalence of gestational diabetes mellitus: a public health perspective. Diabetes Care 2007;30:S141-6. [Crossref] [PubMed]
- Sanchez-Muniz FJ, Gesteiro E, Esparrago Rodilla M, et al. Maternal nutrition during pregnancy conditions the fetal pancreas development, hormonal status and diabetes mellitus and metabolic syndrome biomarkers at birth. Nutr Hosp 2013;28:250-74. [PubMed]
- Ibarra A, Vega-Guedes B, Brito-Casillas Y, et al. Diabetes in Pregnancy and MicroRNAs: Promises and Limitations in Their Clinical Application. Noncoding RNA 2018;4: [Crossref] [PubMed]
- He Y, Bai J, Liu P, et al. miR-494 protects pancreatic beta-cell function by targeting PTEN in gestational diabetes mellitus. EXCLI J 2017;16:1297-307. [PubMed]
- Clausen TD, Mathiesen ER, Hansen T, et al. High prevalence of type 2 diabetes and pre-diabetes in adult offspring of women with gestational diabetes mellitus or type 1 diabetes: the role of intrauterine hyperglycemia. Diabetes Care 2008;31:340-6. [Crossref] [PubMed]
- Osmond DT, Nolan CJ, King RG, et al. Effects of gestational diabetes on human placental glucose uptake, transfer, and utilisation. Diabetologia 2000;43:576-82. [Crossref] [PubMed]
- Casas-Agustench P, Fernandes FS, Tavares do Carmo MG, et al. Consumption of distinct dietary lipids during early pregnancy differentially modulates the expression of microRNAs in mothers and offspring. PLoS One 2015;10:e0117858 [Crossref] [PubMed]
- Dias S, Pheiffer C, Abrahams Y, et al. Molecular Biomarkers for Gestational Diabetes Mellitus. Int J Mol Sci 2018;19: [Crossref] [PubMed]
- Stirm L, Huypens P, Sass S, et al. Maternal whole blood cell miRNA-340 is elevated in gestational diabetes and inversely regulated by glucose and insulin. Sci Rep 2018;8:1366. [Crossref] [PubMed]
- Ebert MS, Sharp PA. Roles for microRNAs in conferring robustness to biological processes. Cell 2012;149:515-24. [Crossref] [PubMed]
- Guay C, Regazzi R. Circulating microRNAs as novel biomarkers for diabetes mellitus. Nat Rev Endocrinol 2013;9:513-21. [Crossref] [PubMed]
- Wang L, Lv Y, Li G, et al. MicroRNAs in heart and circulation during physical exercise. J Sport Health Sci 2018;7:433-41. [Crossref] [PubMed]
- Boon RA, Vickers KC. Intercellular transport of microRNAs. Arterioscler Thromb Vasc Biol 2013;33:186-92. [Crossref] [PubMed]
- Fernandez-Sanjurjo M, de Gonzalo-Calvo D, Fernandez-Garcia B, et al. Circulating microRNA as Emerging Biomarkers of Exercise. Exerc Sport Sci Rev 2018;46:160-71. [Crossref] [PubMed]
- Li J, Zhang Y, Li D, et al. Small non-coding RNAs transfer through mammalian placenta and directly regulate fetal gene expression. Protein Cell 2015;6:391-6. [Crossref] [PubMed]
- Ding R, Guo F, Zhang Y, et al. Integrated Transcriptome Sequencing Analysis Reveals Role of miR-138-5p/ TBL1X in Placenta from Gestational Diabetes Mellitus. Cell Physiol Biochem 2018;51:630-46. [Crossref] [PubMed]
- Gillet V, Ouellet A, Stepanov Y, et al. MicroRNA Profiles in Extracellular Vesicles From Serum Early in Pregnancies Complicated by Gestational Diabetes Mellitus. J Clin Endocrinol Metab 2019; [Epub ahead of print]. [Crossref] [PubMed]
- Li L, Wang S, Li H, et al. microRNA-96 protects pancreatic beta-cell function by targeting PAK1 in gestational diabetes mellitus. Biofactors 2018;44:539-47. [Crossref] [PubMed]
- Nair S, Jayabalan N, Guanzon D, et al. Human placental exosomes in gestational diabetes mellitus carry a specific set of miRNAs associated with skeletal muscle insulin sensitivity. Clin Sci (Lond) 2018;132:2451-67. [Crossref] [PubMed]
- Peng HY, Li HP, Li MQ. High glucose induces dysfunction of human umbilical vein endothelial cells by upregulating miR-137 in gestational diabetes mellitus. Microvasc Res 2018;118:90-100. [Crossref] [PubMed]
- Strutz J, Cvitic S, Hackl H, et al. Gestational diabetes alters microRNA signatures in human feto-placental endothelial cells depending on fetal sex. Clin Sci (Lond) 2018;132:2437-49. [Crossref] [PubMed]
- Wang H, She G, Zhou W, et al. Expression profile of circular RNAs in placentas of women with gestational diabetes mellitus. Endocr J 2019;66:431-41. [Crossref] [PubMed]
- Wang P, Wang H, Li C, et al. Dysregulation of microRNA-657 influences inflammatory response via targeting interleukin-37 in gestational diabetes mellitus. J Cell Physiol 2019;234:7141-8. [Crossref] [PubMed]
- Rahimi G, Jafari N, Khodabakhsh M, et al. Upregulation of microRNA processing enzymes Drosha and Dicer in gestational diabetes mellitus. Gynecol Endocrinol 2015;31:156-9. [Crossref] [PubMed]
- Bianchi C, Battini L, Aragona M, et al. Prescribing exercise for prevention and treatment of gestational diabetes: review of suggested recommendations. Gynecol Endocrinol 2017;33:254-60. [Crossref] [PubMed]
- Zhao Z, Moley KH, Gronowski AM. Diagnostic potential for miRNAs as biomarkers for pregnancy-specific diseases. Clin Biochem 2013;46:953-60. [Crossref] [PubMed]
Cite this article as: Pinto-Hernández P, Tomás-Zapico C, Iglesias-Gutiérrez E. Circulating microRNAs as modifiable diagnostic biomarkers of gestational and transgenerational metabolic risk: can exercise play a role? Non-coding RNA Investig 2019;3:23.