Krüppel-like factor 4-mediated smooth muscle cell phenotype switching to a galectin-3 positive subclass is a detrimental event in the pathogenesis of atherosclerotic cardiovascular disease
Cardiovascular diseases such as coronary artery disease, myocardial infarction and stroke are considered the major cause of death worldwide. Atherosclerosis, narrowing of the arterial lumen due to accumulation of cholesterol within the vessel wall, represents the primary underlying pathology of cardiovascular diseases (1). Cholesterol lowering treatments are currently the first choice therapy for human subjects at risk of developing cardiovascular disease given that hypercholesterolemia is an established risk factor for the development lipid-laden macrophage foam cells, a decisive step in the formation of atherosclerotic lesions. Unfortunately, statin-induced lipid lowering is only able to achieve a 15–25% risk reduction in cardiovascular disease event rate (2,3). As such, there remains a clear need to uncover additional means to battle the occurrence of atherosclerotic cardiovascular disease.
Although cholesterol accumulation in macrophages is a hallmark of atherosclerosis, many other cell types have also been suggested to play a role in the initial development and progression towards more advanced atherosclerotic lesions. These include cells from the innate and adaptive immune system, i.e., T and B cells, neutrophils, and mast cells as well as smooth muscle cells (1). However, the relative contribution of each individual cell type to the atherosclerosis pathology and overall cardiovascular disease outcome is not well understood.
In the paper by Alencar et al. published in the journal Circulation in July 2020 (4), the authors have tried to provide novel insight in the specific role of smooth muscle cells in atherosclerosis. In detail, through combining novel and earlier established gene editing approaches in mice with state-of-the-art single cell RNA sequencing and plaque visualization methods, the authors have tested the hypothesis that smooth muscle cells can obtain different phenotypes within the atherosclerotic lesion context and that the different smooth muscle cell subtypes also differentially impact on the pathology.
Using unbiased RNA sequencing it was found by Alencar et al. that 14 different cell clusters are present in advanced atherosclerotic lesions from both mice and humans and that the smooth muscle cell clusters overlap significantly between the two species with regard to their functional classification (4). Recently published RNA sequencing studies by Depuydt et al. have similarly identified 14 distinct cell populations in human carotid atherosclerotic plaques. They also observed that—besides smooth muscle cells—human atherosclerotic lesions indeed contain endothelial cells, mast cells, B cells, myeloid cells (monocytes/macrophages), and T cells (5).
An interesting aspect of the studies by Alencar et al. was that they could trace back the cellular origin of individual cell clusters through the use of lineage-specific fluorescent reporters. More specifically, it was revealed that 7 out of the total 14 cell populations in murine atherosclerotic plaques are actually derived from smooth muscle cells (4). Only 3 of these 7 specific cell clusters—however—displayed a relative high expression level of the “traditional” smooth muscle cell lineage gene markers alpha-smooth muscle actin/actin alpha 2 (ACTA2), myosin heavy chain 11 (MYH11), or calponin 1(CNN1). It can thus be suggested that lesional smooth muscle cells exhibit a high degree of plasticity, i.e. many smooth muscle cells located within atherosclerotic plaques have lost the expression of these specific markers as this particular smooth muscle cell population shifted its function towards that of other types of cells. In line with this assumption, Alencar et al. detected relatively high expression levels of traditional gene markers for endothelial cells, macrophages, and T cells in certain cell populations that could be traced back to a smooth muscle cell (MYH11-expressing lineage) origin (4). In addition, a significant extent of endothelial to mesenchymal transition seems to occur within plaques as evident from the finding that several endothelial lineage-derived cell populations exhibited relatively high levels of the (vascular) smooth muscle cell markers ACTA2 or vimentin. Notably, these findings corroborate the earlier observation by Allahverdian et al. that ~40% of all CD68-positive macrophages within human coronary artery lesions are derived from smooth muscle cells (6).
The transcription factor Krüppel-like factor 4 (KLF4) has a pro-atherogenic function within smooth muscle cells, since smooth muscle cell-specific gene deletion of KLF4 in mice is associated with a marked reduction in lesion size, and increases in multiple indices of plaque stability (7). The most important insight from the combined imaging, cell isolation and RNA sequencing studies by Alencar et al. was that they also provided novel clues to a possible mechanism driving this pro-atherogenic contribution of KLF4 in smooth muscle cells. More specifically, it was shown that KLF4 expression in smooth muscle cell induces a phenotype shift towards a galectin-3-positive state (4). Galectin-3, encoded by the LGALS3 gene, is a prominent member of the beta-galactoside-binding lectin protein family and known to play a role in many cellular functions including cell-cell adhesion, cell-matrix interactions, transcription regulation, and the control of the cell cycle (8). Recent clinical association studies by Aguilar et al. have shown that high plasma levels of galectin-3 are a predictive marker for the risk of the development of cardiovascular events (9). In addition, higher levels of galectin-3 in serum of a cohort of patients with acute ischemic stroke were independently associated with increased risk of death or major disability after the stroke onset (10). Furthermore, Lisowska et al. have shown that concentrations of galectin-3 are significantly elevated in patients suffering from coronary artery disease than those in non-diseased controls and that people with the highest levels of galectin-3 displayed a significantly increased risk of dying after suffering from a myocardial infarction (11). Galectin-3 is a direct transcriptional target of KLF4 (12). It can therefore be proposed that (pharmacologically) disrupting the interaction of KLF4 with the galectin-3 promoter to inhibit galectin-3 function could be of therapeutic interest to reduce the risk of developing atherosclerotic cardiovascular disease and the associated event mortality. However, it should be acknowledged that blockade of KLF4/galectin-3 function would have to occur in a smooth muscle cell-specific manner, since in macrophages, as opposed to smooth muscle cells, the KLF4-galectin-3 axis rather executes an anti-atherogenic effect (see Figure 1). A selective deficiency in either KLF4 or galectin-3 within macrophages is associated with a more pro-inflammatory state and, as result, a higher susceptibility for the development of atherosclerotic lesions in mice (13,14). In further support of a putative anti-atherogenic effect of galectin-3, an increased (inflammatory) early atherosclerosis burden was detected upon feeding an atherogenic diet in total body galectin-3 knockout mice as compared to pair-fed wild-type mice (15).
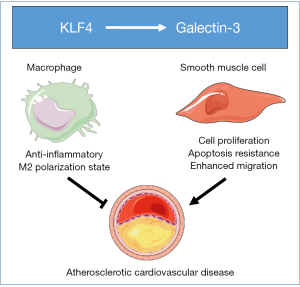
In conclusion, Alencar et al. have highlighted that targeting KLF4-driven plasticity of smooth muscle cells within atherosclerotic lesions could be of therapeutic interest. Furthermore, they spotlight the application potential of their combined single cell RNA sequencing and fluorescent cell lineage-tracing technique to not only (1) globally study cell plasticity in many disease settings, including atherosclerotic cardiovascular disease, but also (2) investigate the potential pathogenic role of individual transcriptional regulators within specific cell lineages.
Acknowledgments
Funding: None.
Footnote
Provenance and Peer Review: This article was commissioned and reviewed by the Section Editor Yujiao Zhu, MD (School of Life Science, Shanghai University, Shanghai, China).
Conflicts of Interest: The author has completed the ICMJE uniform disclosure form (available at http://dx.doi.org/10.21037/ncri-20-7). The author has no conflicts of interest to declare.
Ethical Statement: The author is accountable for all aspects of the work in ensuring that questions related to the accuracy or integrity of any part of the work are appropriately investigated and resolved.
Open Access Statement: This is an Open Access article distributed in accordance with the Creative Commons Attribution-NonCommercial-NoDerivs 4.0 International License (CC BY-NC-ND 4.0), which permits the non-commercial replication and distribution of the article with the strict proviso that no changes or edits are made and the original work is properly cited (including links to both the formal publication through the relevant DOI and the license). See: https://creativecommons.org/licenses/by-nc-nd/4.0/.
References
- Ross R. Atherosclerosis--an inflammatory disease. N Engl J Med 1999;340:115-26. [Crossref] [PubMed]
- Li M, Wang X, Li X, et al. Statins for the Primary Prevention of Coronary Heart Disease. Biomed Res Int 2019;2019:4870350 [Crossref] [PubMed]
- Baigent C, Keech A, Kearney PM, et al. Efficacy and safety of cholesterol-lowering treatment: prospective meta-analysis of data from 90,056 participants in 14 randomised trials of statins. Lancet 2005;366:1267-78. [Crossref] [PubMed]
- Alencar GF, Owsiany KM. The Stem Cell Pluripotency Genes Klf4 and Oct4 Regulate Complex SMC Phenotypic Changes Critical in Late-Stage Atherosclerotic Lesion Pathogenesis. Circulation 2020; [Epub ahead of print]. [Crossref] [PubMed]
- Depuydt MAC, Prange KHM, Slenders L, et al. Microanatomy of the Human Atherosclerotic Plaque by Single-Cell Transcriptomics. Circ Res 2020;127:1437-55. [Crossref] [PubMed]
- Allahverdian S, Chehroudi AC, McManus BM, et al. Contribution of intimal smooth muscle cells to cholesterol accumulation and macrophage-like cells in human atherosclerosis. Circulation 2014;129:1551-9. [Crossref] [PubMed]
- Shankman LS, Gomez D, Cherepanova OA, et al. KLF4-dependent phenotypic modulation of smooth muscle cells has a key role in atherosclerotic plaque pathogenesis. Nat Med 2015;21:628-37. [Crossref] [PubMed]
- Dong R, Zhang M, Hu Q, et al. Galectin-3 as a novel biomarker for disease diagnosis and a target for therapy Int J Mol Med 2018;41:599-614. (Review). [PubMed]
- Aguilar D, Sun C, Hoogeveen RC, et al. Levels and Change in Galectin-3 and Association With Cardiovascular Events: The ARIC Study. J Am Heart Assoc 2020;9:e015405 [Crossref] [PubMed]
- Wang A, Zhong C, Zhu Z, et al. Serum Galectin-3 and Poor Outcomes Among Patients With Acute Ischemic Stroke. Stroke 2018;49:211-4. [Crossref] [PubMed]
- Lisowska A, Knapp M, Tycińska A, et al. Predictive value of Galectin-3 for the occurrence of coronary artery disease and prognosis after myocardial infarction and its association with carotid IMT values in these patients: A mid-term prospective cohort study. Atherosclerosis 2016;246:309-17. [Crossref] [PubMed]
- Ma J, Yao Y, Wang P, et al. MiR-152 functions as a tumor suppressor in glioblastoma stem cells by targeting Krüppel-like factor 4. Cancer Lett. 2014;355:85-95. [Crossref] [PubMed]
- Sharma N, Lu Y, Zhou G, et al. Myeloid Krüppel-like factor 4 deficiency augments atherogenesis in ApoE-/- mice--brief report. Arterioscler Thromb Vasc Biol 2012;32:2836-8. [Crossref] [PubMed]
- Di Gregoli K, Somerville M, Bianco R, et al. Galectin-3 Identifies a Subset of Macrophages With a Potential Beneficial Role in Atherosclerosis. Arterioscler Thromb Vasc Biol 2020;40:1491-509. [Crossref] [PubMed]
- Iacobini C, Menini S, Ricci C, et al. Accelerated lipid-induced atherogenesis in galectin-3-deficient mice: role of lipoxidation via receptor-mediated mechanisms. Arterioscler Thromb Vasc Biol 2009;29:831-6. [Crossref] [PubMed]
Cite this article as: Hoekstra M. Krüppel-like factor 4-mediated smooth muscle cell phenotype switching to a galectin-3 positive subclass is a detrimental event in the pathogenesis of atherosclerotic cardiovascular disease. Non-coding RNA Investig 2020;4:8.