Exosomal microRNAs in cardiovascular diseases
Introduction
MircoRNAs (miRNAs, miRs) are a class of endogenous, 21–25-nucleotide, highly conserved noncoding RNAs that regulate target gene expression by binding messenger RNAs (mRNAs) and inhibiting or reducing translation. A single miRNA can regulate numerous genes, while a single gene can be regulated by multiple miRNAs. At present, hundreds of miRNAs have been identified in human genome, and almost all tissue types have been found to have the expression of miRNAs. MiRNAs have been proved to be involved in the pathological processes of cardiovascular diseases (CVDs), one of the major threats to human health (1). Moreover, miRNAs are identified as novel biomarkers for diagnosis, prognosis, and reaction to therapy for CVDs (2). However, by which means do miRNAs act as regulator in cell-to-cell communication during CVDs is still largely unknown.
Exosomes, one of the most important kinds of extracellular vesicles (EVs), are nanovesicles containing DNA, RNA, proteins, and metabolites of producing cells. It is well-known that exosomes are mediators of proximal as well as distant cell-to-cell signaling (3,4). Interestingly, exosomes are now thought to be the carrier of the circulating miRNAs, which explains why the miRNAs are stable in the enzyme-enriched environment.
In this review, functional roles of exosomal miRNAs in CVD and potential of exosomal miRNAs as novel biomarkers for diagnosis, prognosis, and reaction to therapy for CVDs are summarized.
Exosomal miRNAs in CVD
When myocardial damage occurs as a result of myocardial infarction (MI), some particular miRNAs are thought to be crucially involved in this pathological process. Cardiac miRNAs (miR-1, miR-133, miR-208, and miR-499) are believed to be expressed specifically in the myocardium, and are involved in the survival of cardiomyocytes (5). Several studies have indicated that these miRNAs markedly alter in the condition of myocardium damage (6). In addition, recent observations revealed that exosomes detected after MI are enriched with cardiac miRNAs (7-10). In combination with previous studies into the cardioprotective properties of cardiac miRNAs, exosomes promise to be regulators in anti-apoptotic (miR-133a and miR-499) (11,12) and anti-fibrotic (miR-133a) (13) processes after MI. Aside from cardiac miRNAs, miR-30a was demonstrated to be highly enriched in exosomes from the serum of acute myocardial infarction (AMI) patients. Moreover, this upregulation of exosomal miR-30a could be induced by hypoxia. Transferring of miR-30a between hypoxic cardiomyocytes contributed to the regulation of cardiomyocyte autophagy (14).
Cardiac pathological remodeling, including impaired angiogenesis, ventricular hypertrophy and cardiac fibrosis, often occur after myocardial injury or cardiomyopathy. New evidences indicated that exosomal miRNAs could take part in this process. One important study revealed that miR-21*, a passenger strand microRNA generally considered to be degraded inside the cytoplasm, was transmitted by exosomes from cardiac fibroblasts to cardiomyocytes. The authors also identified that sarcoplasmic protein sorbin and SH3 domain-containing protein 2 (SORBS2) and PDZ and LIM domain 5 (PDLIM5) as targets of miR-21* when released within cardiomyocytes, on which it promotes a hypertrophic response (15). In neonatal rat cardiomyocytes stimulated with Ang II, exosomes were identified to be released from cardiac fibroblasts via activation of both angiotensin II type 1 receptor (AT1R) and angiotensin II type 2 receptor (AT2R), thereby intensifying Ang II-inducing cardiac pathological hypertrophy. Exosomal miR-132 was the core regulator in this paracrine mechanism (16). Aside from cardiac fibroblasts, adipose tissues are now identified as key regulators of cardiac remodeling. Fang et al. found that delivery of miR-200a in adipocyte-derived exosomes to cardiomyocytes resulted in decreased TSC1 and subsequent mTOR activation, leading to cardiomyocyte hypertrophy (17).
Diabetic cardiomyopathy, as a severe complication of diabetes mellitus, results in impaired angiogenesis in the heart. I miR-320 was reported to be enriched in exosomes collected from the spontaneous diabetic rat cardiomyocytes and high glucose-treated cardiomyocytes. In addition, diabetic cardiomyocyte-derived exosomes could inhibit endothelial cell proliferation, migration and tube-like formation by transferring miRNA-320 (18). On the other hand, exercise enhanced exosomes’ release from diabetic hearts, and the exosomal miR-29b and miR-455 could prevent the downstream detrimental effects of MMP9 that lead to fibrosis (19). The role of exosomal miRNAs in pathological progresses of CVDs is summarized in Figure 1.
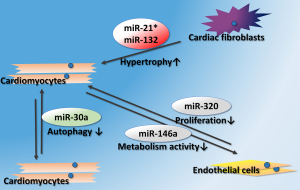
Exosomal miRNAs in treatment of CVD
Mesenchymal stem cells (MSCs) have been reported as a potential therapeutic approach for MI. Now therapeutic potential of MSCs is also identified to be related to exosomes. The first report to provide evidence of exosomal miRNA shedding from MSCs was published in 2014. MicroRNA microarray was used to profile miRNAs in the exosomes of MSCs from mouse bone marrow subjected to ischemic preconditioning. MiR-22 was highly up-regulated in exosomes of MSCs and was demonstrated to be transferred from MSCs to cardiomyocytes and could reduce apoptosis (20). MiR-19a, an anti-apoptotic miRNA, was found to be enriched in exosomes derived from MSCs overexpressing GATA-4. MSCs overexpressing GATA-4 could significantly improve ischemic myocardial function. Further investigations conducted in neonatal rat cardiomyocytes revealed that miR-19a and miR-451 were significantly higher in MSCs overexpressing GATA-4 and inhibition of miR-19a could partially abolish MSCs-mediated cardioprotection by TUNEL assay (21). In a rat model of MI, endometrium-derived MSCs (EnMSCs) demonstrated superior cardioprotective ability relative to bone marrow or adipose-derived MSCs that correlated with enhanced paracrine actions and higher expression of exosomal miR-21. To validate the role for miR-21 in EnMSC-specific paracrine and cardioprotective effects, EnMSCs were transfected with a miR-21 antagonist miR or negative control and employed apoptosis or angiogenesis assays. As a result, cardiomyocytes transfected with miR-21 inhibitor displayed higher apoptotic indexes after hypoxia incubation, while miR-21 knock-down HUVECs had lower microvessel densities, which implied an essential role of exosomal miRNAs in MSCs application for treatment of coronary artery disease (22).
Aside from MSCs, cardiac stem cells (CSCs) can also protect cardiomyocytes via paracrine effects. Exosomes derived from CPCs can protect cardiomyocytes from oxidative stress, and miR-451, one of the GATA4-responsive miRNAs, was enriched in exosomes derived from CPCs (23). In the EVs (most of which were analyzed as exosomes) secreted by human CPCs, miR-210, miR-132, and miR-146a-3p were most highly enriched (24). Recently, more efforts were made for better understanding of the contribution of exosomal miRNAs in CPCs treatment. Exosomes secreted by hypoxic CPCs were loaded with anti-fibrotic and pro-angiogenetic miRNAs, including miR-15b, miR-17, miR-20a, miR-103, miR-199a, miR-210, and miR-29. These miRNAs may be responsible for the tube formation enhancement and pro-fibrotic gene expression decreasing after hypoxic CPC-derived exosomes were applied to ECs and TGF-β-stimulated fibroblasts, respectively (25). In addition, miR-21 was found to be enriched in exosomes secreted from CPCs pretreated under oxidative stress. The exosomal miR-21 induced anti-apoptotic effects on H9c2 cardiomyocytes from oxidative stress (26). Cardiosphere-derived cells (CDCs), one resource of CSCs, can stimulate regeneration and angiogenesis after MI by indirect mechanisms, in which exosomes may be involved. Exosomes secreted by CDCs are critical agents of regeneration and cardioprotection in the AMI mouse model. To investigate the basis of the therapeutic benefit of CDC exosomes, miR-146a was found to be the most highly enriched miRNA in CDCs and exosomal miR-146a reproduced part of the salient benefits of CDCs (27). In addition, several miRNAs, including miR-146a, miR-181b, and miR-126, were enriched in CDCs-secreted exosomes and transfer of miR-181b from CDCs into macrophages preserved cardiac function after MI in the rat model (28).
Application of embryonic stem cells (ESCs) and induced pluripotent stem cells (iPSCs) in MI is another promising strategy against myocardial apoptosis and cardiac function decrease. However, ESCs and iPSCs have the same difficulties in cell retention, coupling and survival in ischemic myocardium when transplanted to the heart. Thus, the cell-free components delivered by ESCs and iPSCs, such as exosomes, maybe an alternative for cardiac protection and regeneration. In a mouse model of AMI, exosomes secreted by ESCs were demonstrated to be able to augment resident CPCs, neo-vascularization, cardiomyocytes proliferation and survival after MI. Moreover, ESCs cell-specific miRNAs, including miR-291, miR-294 and miR-295, were highly enriched in exosomes secreted by ESCs. The effects of ESCs exosomes on CPCs were mimicked partly by treatment of these miRNAs (29). As for iPSCs, reduced activated Caspase3 expression in ischemic myocardium in vivo induced by iPSC-derived exosomes was partly due to the transmission of specific miRNAs such as miR-210 (30). Exosomal miRNAs related to cell therapy for CVDs are summarized in Table 1.
Table 1
miRNA | Cell source | Functions | References |
---|---|---|---|
miR-22 | MSCs | Reduce CMs apoptosis | (20) |
miR-19a | MSCs | Reduce CMs apoptosis | (21) |
miR-21 | EnMSCs | Reduce CMs apoptosis, |
(22) |
miR-451 | CPCs | Reduce CMs apoptosis | (23) |
miR-210, miR-132, and miR-146a-3p | CPCs | Reduce CMs apoptosis, |
(24) |
miRNA-15b, miRNA-17, miRNA-20a, miRNA-103, miRNA-199a, miRNA-210, and miRNA-29 | CPCs | Induce ECs angiogenesis, |
(25) |
miR-21 | CPCs | Reduce CMs apoptosis | (26) |
miR-146a | CDCs | Increased CMs viability, protected against oxidant stress | (27) |
miR-146a, miR-181b, and miR-126 | CDCs | Alter macrophage phenotype | (28) |
miRNA-291, miRNA-294 and miRNA-295 | ESCs | Enhance CPCs survival and function | (29) |
CVD, cardiovascular disease; MSCs, mesenchymal stem cells; EnMSCs, endometrium-derived MSCs; CPCs, cardiac progenitor cells; CDCs, cardiosphere-derived cells; CMs, cardiomyocytes; ECs, endothelial cells.
Remote ischemic preconditioning (RIPC) by repeated treatment of transient limb ischemia is a simple method for protecting heart from reperfusion injury. Exosomes are now believed to be responsible for the transmission of protective stimulus from limbs to the heart. MiR-144 was the first exosomal miRNA reported to play an important role in the cardioprotection induced by RIPC (31). In chronic heart failure (HF) after MI, RIPC significantly attenuated MI-induced fibrosis in the boundary region. Furthermore, exosomal miR-29a was found to be significantly increased in marginal area of the RIPC group (32).
Exosomal miRNAs as biomarkers of CVD
During CVDs, especially in AMI, elevation of cardiac miRNAs is detectable in the serum of patients, making them promising circulating biomarkers for AMI. Until now, there are several reports indicating cardiac miRNAs may be transferred via exosomes. Circulating miR-1 and miR-133a were elevated early in patients with unstable angina pectoris prior to troponin T elevation and were released from infarcted and peri-infarcted myocardium. The release of miR-133a in the exosome fraction from the culture medium of H9c2 cardiomyoblasts was firstly observed, and the release was dose-dependently stimulated by a calcium ionophore (7). Besides, one of the cardiac miRNAs, miR-1 was also found quickly increased in serum exosomes of rats after AMI. Furthermore, fluorescence tracing of labelled exosomes indicated that circulating exosomes could enter the kidney tissues and kidney cells, which provided a possibility for using urine miRNAs as biomarkers for ischemic heart disease such as AMI (8). Earlier, several miRNAs (miRNA-1, -21, -146a, -208, and -499) were also found to have a good diagnostic value for predicting MI in a large cohort of patients with suspected acute coronary syndrome (33). Recently, by miRNA analysis for isolated of plasma-derived EV from porcine model of MI, they further investigated the detailed information regarding the temporal release profile and transportation of these miRNAs in the circulation. It was indicated that some of the circulating or cardiac miRNAs (miRNA-133b, -208b, and -499) were transported by EVs, which were mainly consist of exosomes and microvesicles (9). In addition, miR-1 and miR-133a levels were also found enriched in exosomes released from neutral lipid-loaded HL-1 cardiomyocytes, suggesting that these two miRNAs in serum hold significant promise as clinical indicators of myocardial steatosis (10). It seems that exosomes could serve as a valuable source of cardiac miRNAs for diagnostic purposes, however, none of these studies mentioned above validated their hypotheses on patient data. Moreover, the exosomal trafficking of cardiac miRNAs from the human heart was investigated and concentrations of exosomal miR-1, miR-24, miR-133a and miR-133b from plasma of patients undergoing coronary-artery-by-pass-graft (CABG) were found to be increased at both 24 and 48 h post-surgery, and were positively correlated with the concentration of cardiac troponin I, the “gold standard” biomarker of MI (34).
As for HF, due to a short half-life in serum, cardiac miRNAs are not considered as predicators of future HF development in post-AMI or chronic HF patients. Consequently, exosomal miRNAs whose expression changes can be detected during the development of HF need to be identified urgently. To identify miRNAs with altered expression in accordance with HF development after the convalescent stage of AMI, expression levels of individual miRNAs in sera collected a median of 18 days after AMI onset using a high-throughput array were compared between HF and control groups. miR-192, a p53-responsive miRNA, was the most significantly upregulated in AMI patients with development of ischemic HF. Further, other p53-responsive miRNAs (miR-194 and miR-34a) along with miR-192 were found to be highly enriched in the exosome fraction separated from collected serum (35). The enrichment of HF predicting miRNAs in exosomes may imply a probable mechanism by which miRNAs can last long after initial cell damage. By screening 186 miRNAs, miR-423-5p, miR-320a, miR-22, and miR-92b were found to be the most increased four miRNAs in the serum of HF patients. These authors also defined a “miRNA-score”, which combined the levels of these four miRNAs into a single score, as a more accuracy predictor for HF than any single miRNA alone. Moreover, further evaluation revealed a significant association between the miRNA-score and several important known prognostic parameters (elevated serum BNP levels, a wide QRS, and dilatation of the left ventricle and left atrium) of HF. Finally, the differences in miRNA levels between the HF group and the controls in the exosomal fraction were found to be similar to those seen in the unfractionated serum, and there was no increase in the strength of the difference, which suggested that these HF-predict miRNAs may not covered all by exosomes in human serum (36). Exosomal miR-146a may serve as a highly specific blood biomarker for diagnosis and risk stratification of patients with peripartum heart failure (PPCM) (37). Most recently, in a naturally occurring canine model, some circulating exosomal miRNAs were indicated as responders to HF secondary to myxomatous mitral valve disease (38). Exosomal miRNAs as potential biomarkers for CVDs are summarized in Table 2.
Table 2
miRNAs | Diseases | Speices | Biosample | References |
---|---|---|---|---|
miR-1 and miR-133a | Unstable angina pectoris | Human | Serum | (7) |
miR-1 | Acute MI | Rat | Urine and serum | (8) |
miRNA-133b, -208b, and -499 | MI | Mouse and porcine | Plasma | (9) |
miR-1, miR-24, miR-133a and miR-133b | Patients undergoing CABG | Human | Plasma | (33) |
MiR-192, miR-194 and miR-34a | Ischemic HF | Human | Serum | (34) |
miR-423-5p, miR-320a, miR-22, and miR-92b | HF | Human | Serum | (35) |
miR-146a | PPCM | Mouse and human | Plasma | (36) |
miR-181c and miR-495 | HF secondary to MMVD | Canine | Plasma | (37) |
CVD, cardiovascular disease; MI, myocardial infarction; CABG, coronary artery bypass graft; HF, heart failure; PPCM, peripartum heart failure; MMVD, myxomatous mitral valve disease.
Summary
New evidences reveal the underlying mechanisms that might be helpful to answer important questions during the study of exosomal miRNAs on pathological processes of CVDs. On the other hand, exosomal miRNAs are considered as novel therapeutic approaches and biomarkers for CVD. However, more preclinical and clinical studies are still needed to investigate the potential of exosomal miRNAs as regulators and biomarkers for the diagnosis and treatment of CVD.
Acknowledgments
Funding: None.
Footnote
Conflicts of Interest: Both authors have completed the ICMJE uniform disclosure form (available at http://dx.doi.org/10.21037/ncri.2017.11.04). The authors have no conflicts of interest to declare.
Ethical Statement: The authors are accountable for all aspects of the work in ensuring that questions related to the accuracy or integrity of any part of the work are appropriately investigated and resolved.
Open Access Statement: This is an Open Access article distributed in accordance with the Creative Commons Attribution-NonCommercial-NoDerivs 4.0 International License (CC BY-NC-ND 4.0), which permits the non-commercial replication and distribution of the article with the strict proviso that no changes or edits are made and the original work is properly cited (including links to both the formal publication through the relevant DOI and the license). See: https://creativecommons.org/licenses/by-nc-nd/4.0/.
References
- Li J, Xu J, Cheng Y, et al. Circulating microRNAs as mirrors of acute coronary syndromes: MiRacle or quagMire? J Cell Mol Med 2013;17:1363-70. [Crossref] [PubMed]
- Bei Y, Chen T, Banciu DD, et al. Circulating Exosomes in Cardiovascular Diseases. Adv Exp Med Biol 2017;998:255-69. [Crossref] [PubMed]
- Bei Y, Yu P, Cretoiu D, et al. Exosomes-Based Biomarkers for the Prognosis of Cardiovascular Diseases. Adv Exp Med Biol 2017;998:71-88. [Crossref] [PubMed]
- Xu J, Zhao J, Evan G, et al. Circulating microRNAs: novel biomarkers for cardiovascular diseases. J Mol Med (Berl) 2012;90:865-75. [Crossref] [PubMed]
- Chistiakov DA, Orekhov AN, Bobryshev YV. Cardiac-specific miRNA in cardiogenesis, heart function, and cardiac pathology (with focus on myocardial infarction). J Mol Cell Cardiol 2016;94:107-21. [Crossref] [PubMed]
- Gidlöf O, Andersson P, van der Pals J, et al. Cardiospecific microRNA plasma levels correlate with troponin and cardiac function in patients with ST elevation myocardial infarction, are selectively dependent on renal elimination, and can be detected in urine samples. Cardiology 2011;118:217-26. [Crossref] [PubMed]
- Kuwabara Y, Ono K, Horie T, et al. Increased microRNA-1 and microRNA-133a levels in serum of patients with cardiovascular disease indicate myocardial damage. Circ Cardiovasc Genet 2011;4:446-54. [Crossref] [PubMed]
- Cheng Y, Wang X, Yang J, et al. A translational study of urine miRNAs in acute myocardial infarction. J Mol Cell Cardiol 2012;53:668-76. [Crossref] [PubMed]
- Deddens JC, Vrijsen KR, Colijn JM, et al. Circulating Extracellular Vesicles Contain miRNAs and are Released as Early Biomarkers for Cardiac Injury. J Cardiovasc Transl Res 2016;9:291-301. [Crossref] [PubMed]
- de Gonzalo-Calvo D, van der Meer RW, Rijzewijk LJ, et al. Serum microRNA-1 and microRNA-133a levels reflect myocardial steatosis in uncomplicated type 2 diabetes. Sci Rep 2017;7:47. [Crossref] [PubMed]
- Li X, Wang J, Jia Z, et al. MiR-499 regulates cell proliferation and apoptosis during late-stage cardiac differentiation via Sox6 and cyclin D1. PLoS One 2013;8:e74504 [Crossref] [PubMed]
- Li S, Xiao FY, Shan PR, et al. Overexpression of microRNA-133a inhibits ischemia-reperfusion-induced cardiomyocyte apoptosis by targeting DAPK2. J Hum Genet 2015;60:709-16. [Crossref] [PubMed]
- Castoldi G, Di Gioia CR, Bombardi C, et al. MiR-133a regulates collagen 1A1: potential role of miR-133a in myocardial fibrosis in angiotensin II-dependent hypertension. J Cell Physiol 2012;227:850-6. [Crossref] [PubMed]
- Yang Y, Li Y, Chen X, et al. Exosomal transfer of miR-30a between cardiomyocytes regulates autophagy after hypoxia. J Mol Med (Berl) 2016;94:711-24. [Crossref] [PubMed]
- Bang C, Batkai S, Dangwal S, et al. Cardiac fibroblast-derived microRNA passenger strand-enriched exosomes mediate cardiomyocyte hypertrophy. J Clin Invest 2014;124:2136-46. [Crossref] [PubMed]
- Lyu L, Wang H, Li B, et al. A critical role of cardiac fibroblast-derived exosomes in activating renin angiotensin system in cardiomyocytes. J Mol Cell Cardiol 2015;89:268-79. [Crossref] [PubMed]
- Fang X, Stroud MJ, Ouyang K, et al. Adipocyte-specific loss of PPARγ attenuates cardiac hypertrophy. JCI Insight 2016;1:e89908 [Crossref] [PubMed]
- Wang X, Huang W, Liu G, et al. Cardiomyocytes mediate anti-angiogenesis in type 2 diabetic rats through the exosomal transfer of miR-320 into endothelial cells. J Mol Cell Cardiol 2014;74:139-50. [Crossref] [PubMed]
- Chaturvedi P, Kalani A, Medina I, et al. Cardiosome mediated regulation of MMP9 in diabetic heart: role of mir29b and mir455 in exercise. J Cell Mol Med 2015;19:2153-61. [PubMed]
- Feng Y, Huang W, Wani M, et al. Ischemic preconditioning potentiates the protective effect of stem cells through secretion of exosomes by targeting Mecp2 via miR-22. PLoS One 2014;9:e88685 [Crossref] [PubMed]
- Yu B, Kim HW, Gong M, et al. Exosomes secreted from GATA-4 overexpressing mesenchymal stem cells serve as a reservoir of anti-apoptotic microRNAs for cardioprotection. Int J Cardiol 2015;182:349-60. [Crossref] [PubMed]
- Wang K, Jiang Z, Webster KA, et al. Enhanced Cardioprotection by Human Endometrium Mesenchymal Stem Cells Driven by Exosomal MicroRNA-21. Stem Cells Transl Med 2017;6:209-22. [Crossref] [PubMed]
- Chen L, Wang Y, Pan Y, et al. Cardiac progenitor-derived exosomes protect ischemic myocardium from acute ischemia/reperfusion injury. Biochem Biophys Res Commun 2013;431:566-71. [Crossref] [PubMed]
- Barile L, Lionetti V, Cervio E, et al. Extracellular vesicles from human cardiac progenitor cells inhibit cardiomyocyte apoptosis and improve cardiac function after myocardial infarction. Cardiovasc Res 2014;103:530-41. [Crossref] [PubMed]
- Gray WD, French KM, Ghosh-Choudhary S, et al. Identification of therapeutic covariant microRNA clusters in hypoxia-treated cardiac progenitor cell exosomes using systems biology. Circ Res 2015;116:255-63. [Crossref] [PubMed]
- Xiao J, Pan Y, Li XH, et al. Cardiac progenitor cell-derived exosomes prevent cardiomyocytes apoptosis through exosomal miR-21 by targeting PDCD4. Cell Death Dis 2016;7:e2277 [Crossref] [PubMed]
- Ibrahim AG, Cheng K, Marbán E. Exosomes as critical agents of cardiac regeneration triggered by cell therapy. Stem Cell Reports 2014;2:606-19. [Crossref] [PubMed]
- de Couto G, Gallet R, Cambier L, et al. Exosomal MicroRNA Transfer Into Macrophages Mediates Cellular Postconditioning. Circulation 2017;136:200-14. [Crossref] [PubMed]
- Khan M, Nickoloff E, Abramova T, et al. Embryonic stem cell-derived exosomes promote endogenous repair mechanisms and enhance cardiac function following myocardial infarction. Circ Res 2015;117:52-64. [Crossref] [PubMed]
- Wang Y, Zhang L, Li Y, et al. Exosomes/microvesicles from induced pluripotent stem cells deliver cardioprotective miRNAs and prevent cardiomyocyte apoptosis in the ischemic myocardium. Int J Cardiol 2015;192:61-9. [Crossref] [PubMed]
- Li J, Rohailla S, Gelber N, et al. MicroRNA-144 is a circulating effector of remote ischemic preconditioning. Basic Res Cardiol 2014;109:423. [Crossref] [PubMed]
- Yamaguchi T, Izumi Y, Nakamura Y, et al. Repeated remote ischemic conditioning attenuates left ventricular remodeling via exosome-mediated intercellular communication on chronic heart failure after myocardial infarction. Int J Cardiol 2015;178:239-46. [Crossref] [PubMed]
- Oerlemans MI, Mosterd A, Dekker MS, et al. Early assessment of acute coronary syndromes in the emergency department: the potential diagnostic value of circulating microRNAs. EMBO Mol Med 2012;4:1176-85. [Crossref] [PubMed]
- Emanueli C, Shearn AI, Laftah A, et al. Coronary Artery-Bypass-Graft Surgery Increases the Plasma Concentration of Exosomes Carrying a Cargo of Cardiac MicroRNAs: An Example of Exosome Trafficking Out of the Human Heart with Potential for Cardiac Biomarker Discovery. PLoS One 2016;11:e0154274 [Crossref] [PubMed]
- Matsumoto S, Sakata Y, Suna S, et al. Circulating p53-responsive microRNAs are predictive indicators of heart failure after acute myocardial infarction. Circ Res 2013;113:322-6. [Crossref] [PubMed]
- Goren Y, Kushnir M, Zafrir B, et al. Serum levels of microRNAs in patients with heart failure. Eur J Heart Fail 2012;14:147-54. [Crossref] [PubMed]
- Halkein J, Tabruyn SP, Ricke-Hoch M, et al. MicroRNA-146a is a therapeutic target and biomarker for peripartum cardiomyopathy. J Clin Invest 2013;123:2143-54. [Crossref] [PubMed]
- Yang VK, Loughran KA, Meola DM, et al. Circulating exosome microRNA associated with heart failure secondary to myxomatous mitral valve disease in a naturally occurring canine model. J Extracell Vesicles 2017;6:1350088 [Crossref] [PubMed]
Cite this article as: Shi J, Kong X. Exosomal microRNAs in cardiovascular diseases. Non-coding RNA Investig 2017;1:19.